Журнал эволюционной биохимии и физиологии, 2023, T. 59, № 5, стр. 345-360
Роль цАМФ в топографической организации обонятельной системы
Е. В. Бигдай 1, А. А. Разинова 1, *
1 Санкт-Петербургский государственный педиатрический медицинский университет
Министерства здравоохранения Российской Федерации
Санкт-Петербург, Россия
* E-mail: annichok@mail.ru
Поступила в редакцию 25.04.2023
После доработки 05.07.2023
Принята к публикации 21.07.2023
- EDN: KKSTPE
- DOI: 10.31857/S0044452923050030
Аннотация
В статье анализируются данные литературы, посвященные роли молекулярных обонятельных рецепторов и цАМФ в формировании топографической организации обонятельной сенсорной системы. Сенсорная информация до передачи ее в мозг организована уже в периферическом отделе по принципу “один нейрон–один рецептор”, распространяющийся и на гломерулы в обонятельной луковице (ОЛ), которые подчиняются закону “одна гломерула–один рецептор”. В настоящее время важную роль в формировании сенсорной карты стали приписывать обонятельным рецепторам, играющим двойную роль в организации обонятельной системы, поскольку локализуются как в обонятельных жгутиках, так и в мембране конуса роста аксона одного и того же обонятельного сенсорного нейрона (ОСН), и определяют мишени для аксонов ОСН в ОЛ. Вместе с тем существуют убедительные доказательства центральной роли внутриклеточной сигнальной системы цАМФ в развитии сенсорной карты. Методом генетической мутации с отменой синтеза цАМФ выявили, что аксоны, несущие эту мутацию, никогда не проникают в гломерулярный слой, а остаются в слое обонятельного нерва. При этом аксоны ОСН нацеливаются на ОЛ, но не могут сформировать отчетливые и четко определенные гломерулы, многие из которых становятся гетерогенными, поскольку содержат волокна, принадлежащие ОСН, экспрессирующим обонятельные рецепторы к разным одорантам. Таким образом, цАМФ, синтезируемый в кончике аксона ОСН, под действием сигналов из ОЛ регулирует экспрессию молекул его навигации к своей мишени в ОЛ, а также образует интрабульбарные химические и электрические синапсы, формируя нейронные цепи. Многочисленные клинические и экспериментальные данные позволили заключить, что патогенетические механизмы развития некоторых психических заболеваний связаны с нарушением регуляции цАМФ.
Список сокращений
цАМФ – циклический аденозинмонофосфат
ОР – обонятельный рецептор
ОЛ – обонятельная луковица
ОЖ – обонятельные жгутики
ОСН – обонятельные сенсорные нейроны
ОЭ – обонятельный нейроэпителий
АЦ – аденилатциклаза
CNG-каналы – цикло-нуклеотид зависимые каналы
ПК-А – протеинкинала А
PEBP1 – фосфатидилэтаноламин-связывающий белок 1
ФДЭ – фосфодиэстераза
ВВЕДЕНИЕ
Восприятие запаха осуществляется ОСН, расположенными в обонятельном нейроэпителии (ОЭ). ОСН экспрессируют специфический для данной пахучей молекулы (одоранта) ОР. ОСН с ОР для одного и того же одоранта располагаются по поверхности ОЭ в одной из зон, внутри которой они перемешиваются с клетками, экспрессирующими ОР к другим пахучим молекулам, в случайном порядке. Строгий топографический порядок устанавливается в ОЛ. Аксоны ОСН проецируются в определенные области ОЛ (гломерулы), где формируются синапсы с постсинаптическими клетками. Причем аксоны ОСН, в которых локализуются ОР к одному и тому же одоранту, посылают проекции на одну и ту же гломерулу, формируя дискретную сенсорную карту [1–3]. Таким образом, на уровне ОЛ образуются нейронные сети, связывающие между собой ОСН с гломерулами, а также разные гломерулы между собой и ОЛ с центрами более высокого порядка. Факт того, что аксоны, принадлежащие обонятельным клеткам, несущим одинаковые ОР, конвергируют на специфические гломерулы в ОЛ и формируют таким образом топографическую сенсорную карту, установлен давно. Вместе с тем в настоящее время активно изучаются специфические молекулярные механизмы навигации аксонов к своим мишеням, в которых важную роль выполняют ОР, локализованные в мембране аксонного холмика ОСН, а также цАМФ, синтезируемый в этой области клетки и регулирующий процесс хемотаксиса аксонов. Поэтому важно знать, какие процессы активируются в кончике аксонов, которые регулируют синтез рецепторов к молекулам наведения с участием ОР и цАМФ.
1. ХАРАКТЕРИСТИКА ОБОНЯТЕЛЬНЫХ СЕНСОРНЫХ НЕЙРОНОВ
Обонятельная система представляет собой очень древнюю и эволюционно высококонсервативную систему. У наземных млекопитающих пахучие молекулы (одоранты) представляют собой летучие, небольшие (как правило, с молекулярной массой менее 400 Да) и преимущественно органические молекулы, растворенные в воздухе [4]. В водной среде молекулы пахучих веществ в основном представляют собой аминокислоты [2, 3].
Одоранты воспринимаются обонятельными клетками, расположенными в основном в ОЭ. В его составе помимо рецепторных имеются базальные и опорные клетки [5]. ОСН являются первичночувствующими (нейросенсорными) рецепторами. От апикального полюса биполярной обонятельной клетки отходит дендрит длиной 20–90 мкм и диаметром 0.8–4.0 мкм, который простирается до апикальной поверхности ольфакторного эпителия. На вершине он образует утолщение – булаву, снабженную ОЖ. В них экспрессируются ОР, которые связываются с одорантами и запускают передачу электрохимического сигнала. От базального полюса ОСН начинается тонкий немиелинизированный и неразветвленный аксон, толщиной 0.1–0.3 мкм. Он, образуя обонятельный нерв, связывающий рецепторные клетки с первичными обонятельными центрами, проецируется непосредственно в ОЛ, место начальной обработки обонятельной информации [4].
2. ОБОНЯТЕЛЬНЫЕ РЕЦЕПТОРЫ
Согласно современной теории обоняния восприятие запаха начинается с взаимодействия пахучей молекулы с молекулярным ОР, преобразующим химические раздражители из внешнего мира в электрические сигналы. В 1991 г. Buck и Axel клонировали и охарактеризовали подгруппу мультигенного семейства ОР, связанных с G-белками (GPCR), экспрессия которых была ограничена ОЭ [6–9]. ОР экспрессируются на клеточной поверхности ОЖ, концентрируясь в их дистальной части, и распознают размер, форму и функциональную группу одоранта [10–13].
Все больше накапливается данных в пользу гипотезы о том, что каждый ОСН экспрессирует только один тип ОР из репертуара более чем 1000 генов ОР [11]. Считается, что механизмом, который обеспечивает экспрессию одного типа ОР в каждом ОСН, является взаимоисключающая и моноаллельная экспрессия рецепторных генов. В результате такого генетического паттерна экспрессии ОР возникает правило: “один ОСН – один ОР”. Как было показано методом in situ гибридизации, ОР экспрессируются в обонятельных клетках грызунов уже на ранней стадии развития (Е12) независимо от созревания клетки, когда периферический отросток еще не прорастает к апикальной поверхности обонятельной клетки, и присутствуют в ней задолго до возникновения обнаружения запаха [14]. Но выбранный паттерн экспрессии данного рецептора сохраняется на протяжении всей жизни клетки [2, 3].
Вместе с тем у позвоночных значительная часть семейства генов для ОР является псевдогенами. На них у гоминидов приходится около 50%, у мышей и собак – 20%, а у приматов – 25–35% генов ОР [13]. Вероятно, улучшение зрительных возможностей сделало обоняние частично излишним, приводя к инактивации функциональных генов [15]. Кроме того, эти различия в рецепторных псевдогенах, по-видимому, отражают поведенческие и экологические различия обонятельных стимулов среди видов [2, 3].
Следует отметить еще одну функцию ОР. Показано, что в ОСН мыши они вовлекаются в механизм реакции на механическое воздействие – ранее неизвестный механизм механотрансдукции. Полагают, что ОР, связанные с G-белком, обладают функцией механических датчиков, которые устанавливают молекулярный механизм, посредством которого афферентный сигнал, связанный с дыханием, поступает из полости носа к мозгу, чтобы синхронизировать активность дельта/тета-диапазона в определенных участках мозга с дыханием [16]. Таким образом, ОР могут быть полимодальными, выполняя роль не только химических, но и механических датчиков. Следовательно, в обонятельной сенсорной системе может кодироваться как информация о запахе, так и о воздушном потоке, которая поступает от ОСН, которые экспрессируют рецепторы с разной чувствительностью к запахам и механическим раздражителям [16, 17].
3. МЕХАНИЗМ ОБОНЯТЕЛЬНОЙ ТРАНСДУКЦИИ
Сигнальный каскад, запускаемый запахами, хорошо охарактеризован (рис. 1). Одоранты, связываясь с ОР в мембране ОЖ ОСН, активируют каскад обонятельной трансдукции, который осуществляется через сопряженный с ольфакторным рецептором G-белком [18]. В ОЭ идентифицирован специфический для обоняния Golf-белок. ОЖ обогащены им главным образом в дистальной части, и, хотя его свойства сходны со стимуляторными G-белками в других тканях, он является особой разновидностью для ОЭ. Golf-белок обладает уникальной для ольфакторного G-протеина конфигурацией ГТФ-связывающего сайта, а его альфа-субъединица способна стимулировать аденилатциклазу (АЦ) [2, 13, 19–21].
Рис. 1.
Схема обонятельной трансдукции. Схема ОСН и схема цАМФ-опосредованного каскада трансдукции в мембране обонятельных жгутиков.
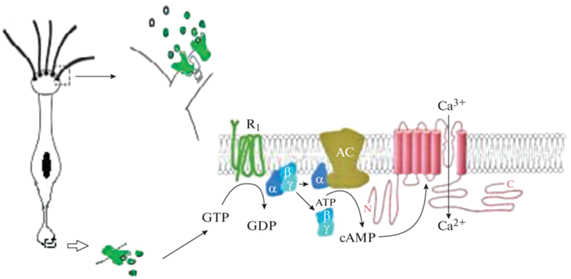
Golf представляет собой периферический белок цилиарной плазматической мембраны, гетеротример, состоящий из альфа-, бета- и гамма-субъединиц [22, 23].
Белком-мишенью для Golf-белка в каскаде ольфакторной трансдукции является аденилатциклаза. У млекопитающих клонировано и охарактеризовано около 9 близкородственных изоформ аденилатциклаз (АЦ). В ОЖ помимо АЦ 3-го типа (АЦ-3) экспрессируются АЦ 2-го типа (АЦ-2) и АЦ 4-го типа (АЦ-4) [24, 25]. Они активируются различными (непахучими) стимулами. Однако именно АЦ-3 является ключевым ферментом, участвующим в продукции циклического аденозинмонофосфата в обонянии. Впервые ее обнаружили именно в ОЭ, где она локализуется в ОЖ [26]. Активированная АЦ-3 гидролизует АТФ, синтезируя цАМФ (рис. 1) [18, 27–30].
Вместе с тем роль АЦ-3 не сводится к прямому участию в процессе восприятия запаха в качестве компонента механизма обонятельной трансдукции. Свое влияние на обонятельную рецепцию этот фермент способен оказывать косвенным путем, поддерживая структуру ОЖ. В частности, опыты на нокаутных мышах обнаружили, что АЦ-3 вовлекается в регуляцию длины ОЖ, и удаление АЦ-3 нарушает целостность цилиарной структуры, модулируя жгутиковую архитектуру в ОСН [31]. Таким образом, помимо основного компонента в механизме обонятельной трансдукции АЦ-3 играет незаменимую роль в постнатальном созревании ОСН и поддержании ультраструктуры ОЖ у грызунов в течение всего взрослого периода [32, 33]. Дефекты в структуре или функции ОЖ приводят к тяжелым сенсорным нарушениям, проявляющимся в аносмии. Поэтому для терапевтического лечения обонятельных нарушений важно понимать, как в ОСН регулируются цилиарная архитектура (т.e. длина и/или форма) и функция [34, 35]. Одним из таких приемов может быть, вероятно, регуляция активности АЦ-3.
В процессе обонятельной трансдукции в результате гидролиза АТФ синтезируется цАМФ (рис. 1). Он получил характеристику вездесущего вторичного мессенджера, поскольку выполняет разнообразные функции в клетках. Этот циклонуклеотид способен регулировать большое количество клеточных процессов, включая важные механизмы поддержания клеточного гомеостазиса, активность различных ферментов, а также ряд специфических функций, связанных с сокращением и расслаблением мышц, секрецией желез, пластичностью нейронов, иммунным ответом, клеточной пролиферацией и апоптозом [36–40]. В ОСН цАМФ в зависимости от своей концентрации может определять морфологию и сохранение ОЖ. Большую роль при этом играет способность цАМФ поддерживать базальные внутрицилиарные уровни (0.1–0.3 мкм) в отсутствие обонятельной стимуляции [41]. Полагают, что базальная продукция цАМФ связана с последующей транскрипцией генов, сопряженных со жгутиками, что, в свою очередь, регулирует их морфологию, а следовательно, и механизм обонятельной трансдукции [31].
Из вышеизложенного следует, что цАМФ обладает плейотропными эффектами. Как в таком случае достигается специфичность передачи сигналов в различных типах клеток, включая ОСН, способная вызывать характерный и соответствующий клеточный ответ? Поскольку константа диффузии цАМФ находится в диапазоне 270–780 мкм2/с, цАМФ считался мессенджером дальнего действия, способным распространяться на большие расстояния и передавать информацию в отдаленные места. В настоящее время считают, что этот циклический нуклеотид действует в пределах отдельных субклеточных доменов, которые ограничивают его диффузию [42, 43].
В обонятельной трансдукции цАМФ выполняет миссию внутриклеточного мессенджера, и его дефицит приводит к многообразным нарушениям обонятельной функции [44]. Это означает, что для поддержания нормального процесса восприятия запаха необходимо регулировать уровень внутрицилиарного цАМФ, который зависит как от его синтеза, так и от деградации (рис. 1). Как известно, функцию поддержания внутриклеточных градиентов цАМФ выполняет фосфодиэстераза (ФДЭ), которая так же, как АЦ-3 и цАМФ, вовлекается в механизм обонятельной трансдукции [42, 45].
Таким образом, в ходе обонятельной рецепции стимуляция Golf-белка, сопряженного с ОР, активируя АЦ-3, продуцирует цАМФ. Увеличение его содержания деполяризует рецепторные нейроны посредством открытия циклонуклеотид-зависимых каналов (CNG-каналов) в цилиарной мембране. Было показано, что они играют решающую роль в обонянии. В результате их открытия входящий ток ионов кальция инициирует Ca2+-зависимый ток Cl-, усиливающий деполяризацию, вызванную Са2+. Результирующая деполяризация генерирует локальный рецепторный потенциал, способный с декрементом распространяться к аксону ОСН [17, 46].
Механизм усиления сигнала имеет важное значение для увеличения обонятельной чувствительности, ведь в интактных обонятельных жгутиках продукция цАМФ невелика (максимально около 200 000 молекул на стимул на клетку). Это означает, что обонятельные клетки обладают низким усилением в энзиматическом каскаде, невыгодным для сигнальной трансдукции, но этот уникальный механизм, как полагают, необходим для снижения потерь АТФ. Однако образование небольшого количества вторичных посредников в обонятельных клетках не уменьшает эффективности процесса внутриклеточной сигнализации. С одной стороны, большой вклад вносят ОЖ, имеющие высокое отношение объема к поверхности. За счет жгутиков плотность рецепторной поверхности ОЭ у быка составляет 20 см3/см2. С другой стороны, в обонятельную трансдукцию вовлекается нелинейное усиление благодаря последовательному открытию CNG-каналов и Са2+-зависимых Cl–каналов, используя Са2+ в качестве третичного посредника [47].
Возникающий в дистальной области ОЖ рецепторный потенциал, распространяясь по ОСН, генерирует потенциал действия в аксонном холмике ОСН, и нервные импульсы распространяются в ОЛ. Аксоны ОСН образуют прямые проекции из ОЭ в ОЛ, где они конвергируют на гломерулы. Из ОЛ аксоны нейронов второго порядка посылают прямые проекции в ипсилатеральную пириформную и энторинальную кору, миндалину, гиппокамп и орбитофронтальную кору.
4. ТОПОГРАФИЯ ОБОНЯТЕЛЬНОЙ СЕНСОРНОЙ СИТЕМЫ
Ринотопическая карта в ОЭ
У грызунов в основном ОЭ лишь малая часть от всей популяции ОСН реагирует на один и тот же одорант. Так, исследования с помощью in situ гибридизации показали, что как у рыб, так и у грызунов, ген каждого ОР экспрессируется в небольшой клеточной фракции. Три различных субсемейства генов для ОР обнаружены только в 0.1–0.2% клеток [48]. На изолированных обонятельных клетках тритона методом пэтч-клэмп выявили, что из 1569 тестируемых клеток только 1.6% реагировали на лилиаль, а из 896 ОСН только 1.7% – на лираль. Из общего числа рецепторных клеток ОЭ клетки, чувствительные к лиралю и лилиалю, занимают около 2% [49]. Это означает, что рецепторные нейроны, реагирующие на один и тот же запах, занимают небольшую часть от всех рецепторных клеток в составе ОЭ. Методами генетического анализа при гибридизации in situ с использованием зондов, специфических к ОР, в эпителии носа крысы были топологически идентифицированы обонятельные клетки, специфически экспрессирующие определенный подтип мембранного рецептора [50].
Топографически группы сенсорных нейронов, экспрессирующих один и тот же ОР, располагаются в больших, но ограниченных зонах вдоль дорсомедиальной и вентролатеральной эпителиальной оси. Внутри одной зоны ОСН, экспрессирующие разные ОР, смешиваются [2, 3], образуя пространственные группы. В этих зонах выделяют дополнительные ОР I и II класса, причем ОР II класса экспрессируются по всей поверхности ОЭ, тогда как экспрессия ОР I класса ограничивается дорсальной частью ОЭ [1]. То есть, обонятельные клетки, имеющие ген одного и того же рецептора, окружены обонятельными клетками с геном другого ОР, и эти группы не перемешиваются между зонами. В результате уже на уровне ОЭ создаются условия, обеспечивающие в дальнейшем проецирование аксонов, принадлежащих ОСН с ОР к данному одоранту, в определенную зону ОЛ. Следовательно, на поверхности ОЭ рецепторные клетки образуют “пространственные карты запахов” [48]. Таким образом, сенсорная информация до передачи ее в мозг организована в периферическом отделе, поскольку определенной зоной ограничены рецепторные клетки, реагирующие на свой специфический пахучий стимул [51, 52].
Таким образом, ОЭ представляет собой мозаику ОР, реагирующих на различные одоранты. Экспрессия ОР является непрерывной и перекрывается в различных зонах по всей дорсомедиальной и вентролатеральной оси ОЭ [2, 3]. При этом ОЖ разных клеток могут перекрываться. Поэтому в пределах одной зоны цилиарная сеть может содержать сложный ассортимент ОР, принадлежащих различным ОЖ.
Топография обонятельной луковицы
Как было отмечено, уже на уровне ОЭ создаются условия, обеспечивающие проецирование аксонов, принадлежащих ОСН с ОР к данному одоранту, в определенную зону ОЛ. В результате в ней достигается пространственный порядок (рис. 2). Здесь аксоны нейронов, экспрессирующих один и тот же ОР, конвергируют, образуя синапсы с постсинаптическими клетками в гломерулах на медиальной и латеральной стороне каждой ОЛ. Конвергенция подобных аксонов с образованием гломерул в ОЛ была напрямую визуализирована посредством направленного мутагенеза на генетически модифицированной линии мышей P2-IRES-Tau-LacZ. У этой линии эндогенный P2-ОР коэкспрессировался с репортерным геном Tau-lacZ, в результате легко идентифицировались P2-нейроны в ОЭ и соответствующих гломерулах в ОЛ [53]. В последующих исследованиях замена Tau-LacZ зеленым флуоресцентным белком (GFP) позволила напрямую визуализировать ОСН и соответствующие гломерулы [2]. Таким образом, на уровне ОЛ происходит пространственное разделение сенсорных афферентов, организуя обонятельную топографическую карту, которая определяет качество и интенсивность сенсорных стимулов [54]. А принцип “один нейрон–один рецептор” распространяется и на гломерулы, которые подчиняются закону “одна гломерула–один рецептор”. В результате такой организации отличительной чертой зрелых гломерул является то, что они образованы исключительно волокнами ОСН, экспрессирующими один и тот же ОР. Такие гломерулы обозначают как гомогенные клубочки [52, 55].
Рис. 2.
Ринотопическая карта в ОЭ (olfactory epithelium). Проекции ОСН (olfactory sensory neurons) на ОЛ (olfactory bulb). ОСН (olfactory sensory neurons), экспрессирующие данный ОР (обозначены каплями одинакового цвета), ограничены одной из частично перекрывающихся зон, в которых ОЭ делится вдоль дорсо-вентральной (DV) оси. Внутри каждой зоны ОСН (olfactory sensory neurons), экспрессирующие разные ОР (обозначены каплями разных цветов), перемешаны. ОСН (olfactory sensory neurons), экспрессирующие ОР того же типа, образуют гломерулы (заштрихованные круги того же цвета, что и соответствующие ОСН (olfactory sensory neurons)) в специфических локусах ОЛ (olfactory bulb). Имеется соответствие между зональной организацией эпителия по ходу дорсо-вентральной (D-V) оси [2].
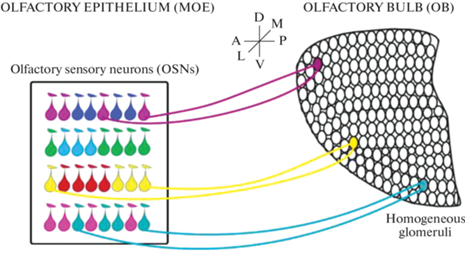
Гломерулу определяют как функциональную единицу, которая обрабатывает сенсорную информацию, связанную с тем ОР, который экспрессируется в ОСН, посылающим свой аксон в данную гломерулу. Эти функциональные единицы образуют “обонятельные колонки”, в которых пространственная сегрегация сенсорных афферентов формирует топографическую карту OЛ (рис. 2) [1, 2, 56, 57].
В экспериментах по функциональной визуализации выявили, что одорант кодируется пространственным паттерном активированных гломерул в соответствии с архитектурой топографической карты и характером комбинаторного кода [58, 59]. Следовательно, для правильного кодирования запахов необходимы конкретное расположение и организация гломерул на карте OЛ (рис. 2). А это, в свою очередь, связано с тем, что каждый клубочек получает информацию только от одного типа ОР, и его спектр реакций на запах определяется лигандсвязывающими свойствами этого рецептора в ОСН [1]. Из этого следует, что гломерула является центральным представителем различных ОР с высокой специфичностью в организации ольфакторных проекций и функциональной специализацией. ОР занимают определенные области в ОЛ и тесно вовлекаются в механизмы формирования этого паттерна проекции. Полагают, что ОР обеспечивают навигацию конуса роста аксонов в ОЛ [53, 60].
Поскольку ОСН, которые экспрессируют один и тот же рецептор, с исключительной точностью конвергируют к специфическим клубочкам на поверхности ОЛ: один на медиальной и один на латеральной стороне каждой ОЛ, пространственный порядок в ней достигается быстро. Этот паттерн конвергенции аксонов формирует анатомическую основу обонятельной сенсорной карты [11].
Таким образом, информация о молекуле одоранта обрабатывается локальными нейрональными цепями, в которых участвуют синаптические взаимосвязи внутри гломерулы, а также между ними в ОЛ. Образованное внутрибульбарное звено обеспечивает второй уровень топографии ОЛ [2, 3].
Из вышесказанного следует: фундаментальным шагом в обонятельном кодировании считают преобразование молекулярных характеристик одоранта в пространственную карту активности нейронов. Суть процесса кодирования информации о запахе заключается в том, что вызываемая пахучим стимулом активность рецепторных нейронов, распределенных по всему ОЭ, трансформируется в пространственно организованный паттерн входа в гломерулы ОЛ. Далее эта карта ввода, вероятно, преобразуется синаптической обработкой внутри клубочков и между ними по мере того, как обонятельная информация передается на нейроны второго порядка (выходные) в ОЛ [59]. Полагают, что изменение эффективности дендро-дендритических синаптических соединений между специфическими подгруппами митральных и пучковых клеток может быть одним из основных механизмов обонятельной памяти.
5. МЕХАНИЗМЫ, ЛЕЖАЩИЕ В ОСНОВЕ ФОРМИРОВАНИЯ СЕНСОРНОЙ КАРТЫ
Роль обонятельных рецепторов
В настоящее время на основе серий генетических экспериментов важную роль в формировании сенсорной карты стали приписывать ОР. Было показано, что для создания топографической карты качества запаха ольфакторные нейроны, экспрессирующие данный ОР, посылают свои проекции точно к 2 из 1800 гломерул в ОЛ. Но делеции или non-sense мутации в гене ОР Р2 приводят к блужданию аксонов этих клеток. Они отклоняются от своего маршрута, а не сходятся на конкретной гломеруле. При замещении гена P2 кодирующей областью гена P3 приводят к проекции аксонов P3 → P2 на гломерулу клубочкового слоя Р3 дикого типа. Следовательно, изменения последовательности ОР приводят к изменению конвергенции сенсорных нейронов и нарушению сенсорной карты [51, 61]. Таким образом, рецептор одоранта играет информативную роль в установлении топографической карты.
Согласно современным представлениям, одни и те же ОР локализуются как в ОЖ ОСН, так и в мембране конуса роста аксона этого рецепторного нейрона. Доказательством этого послужили иммуногистохимические исследования и исследования с двойным мечением с использованием линий трансгенных мышей. На серийных срезах ОЛ линий mOR37-трансгенных мышей эксперименты с двойной меткой показали, что отдельные иммунореактивные клубочки соответствуют гломерулам, на которые нацелены нейроны, экспрессирующие определенный член подсемейства рецепторов mOR37. Эти данные указывают на то, что белки ОР действительно присутствуют в аксональных отростках и ОЖ обонятельных нейронов. Таким образом, получены убедительные подтверждения представлений о том, что ОР участвуют в молекулярных процессах, лежащих в основе нацеливания обонятельных аксонов на ОЛ. Следовательно, ольфакторные рецепторы, расположенные в разных областях обонятельной клетки, обладают разными функциями: обнаруживают запахи в ОЭ и обеспечивают электрохимическое сопряжение для последующей генерации потенциалов действия, распространяющихся в ОЛ, тогда как эти же ОР на аксональном конусе роста, определяют мишени для аксонов ОСН в ОЛ [62].
Конус роста ОСН рассматривают как автономный компартмент, где локально транслируются и экспрессируются молекулы, участвующие в навигации аксона, наделяя окончание аксона способностью быстро реагировать на сигналы, встречающиеся на пути к его цели. Поэтому уникальная особенность организации обонятельной системы состоит в том, что ОР в ней играют двойную роль [2, 3, 63].
Механизм, лежащий в основе роли ОР в навигации аксона, изучен недостаточно. В одной из моделей предполагают, что ОР функционирует как рецептор наведения аксонов, способный распознавать молекулярные сигналы, происходящие из клеток в ОЛ, предоставляя аксону информацию для хемотаксиса. Согласно другой модели, для конвергенции сходных аксонов с образованием клубочков в специфических локусах в ОЛ в первую очередь необходимо создать взаимодействие между аксонами, экспрессирующими один и тот же ОР (гомотипическими аксонами). Полагают, что обе эти модели могут сосуществовать, но, возможно, на разных этапах процесса регуляции аксонального наведения. На разных стадиях могут доминировать разные механизмы [11].
Сигнальный путь, связанный с цилиарными ОР, хорошо известен. Он вовлекает цАМФ и Ca2+. Функциональные характеристики ОР на аксональных конусах роста стали исследовать гораздо позже. Пространственно-временной анализ динамики цАМФ и Ca2+ в ОСН in vitro и in situ выявил, что ольфакторные рецепторы в конусе роста, так же, как и в ОЖ, способны связывать запахи и активировать аналогичный механизм трансдукции, вовлекающий стимуляцию АЦ-3, синтез цАМФ, который обеспечивает приток Ca2+ через CNG-каналы. Однако в этой зоне ОСН ионы кальция включаются в процесс экзоцитоза медиатора глутамата, а локальное повышение цАМФ инициирует активацию и транслокацию протеинкиназы А (ПК-А) в ядро. Эти данные свидетельствуют также о том, что Ca2+ и цАМФ, связанный с ОР аксонов, может оказывать свое действие в двух разных местах. Во-первых, локально, регулируя динамику цитоскелета для модуляции удлинения и поворота конуса роста аксона. Во-вторых, в ядре, посредством активации ПK-A для регулирования экспрессии молекул, участвующих в процессе наведения аксонов [11, 64]. Таким образом, были определены функциональные свойства ОР на конусе роста. Они играют роль в конвергенции аксонов и формировании сенсорной карты.
Эти данные свидетельствуют о том, что цАМФ, образующийся в результате активации ОР в аксонах, может оказывать свое действие в двух разных местах: локально, в области пресинапса, и в ядре [65]. Полагают, что локальный синтез цАМФ, инициируемый аксональными ОР, указывает на роль цАМФ в регуляции наведения аксонов, поскольку Gs-опосредованные сигналы цАМФ регулируют транскрипцию генов, кодирующих молекулы наведения аксонов, которые, в свою очередь, определяют расположение клубочков. Известно, что эти вторичные мессенджеры играют ключевую роль в удлинении и повороте аксонов в нескольких системах, включая обонятельную [26, 33, 66].
Топографическое строение ОЛ требует четкого соответствия между ОСН, экспрессирующими данный ОР, со специфической гломерулой в ольфакторной луковице. Таким образом, формирование обонятельной карты зависит от тесной координации между экспрессией определенных навигационных молекул и определенных ОР. Однако механизм активации и функции рецептора запаха на конце аксона до сих пор неизвестен. Полагают, что существуют различные, специфические для мишени наборы рецепторов наведения, экспрессируемых в каждой категории нейронов [67]. Утверждают, что аксональные ОР могут действовать как сигналы наведения аксонов, активируясь молекулами, экспрессируемыми в ОЛ. Первым предполагаемым лигандом для них методом масс-спектрометрии был идентифицирован фосфатидил-этаноламин-связывающий белок 1 (PEBP1). PEBP1 представляет собой небольшую молекулу (около 21 кДа), экспрессирующуюся в нейронах и ненейрональных клетках во многих областях мозга [61, 68]. В ОЛ мышей и крыс PEBP1 локализуется в основном в перигломерулярных клетках, взаимодействующих с входящими сенсорными аксонами. Свидетельством того, что PEBP1 является лигандом для аксональных ОР, послужили данные, в которых было показано, что его аппликация на кончик аксона ОСН и клетки HEK, экспрессирующих специфические ОР, вызывала в этой области увеличение содержания Ca2+. Однако несмотря на то, что получены убедительные доказательства непосредственного участия аксональных ОР в навигации аксонов ОСН к их мишени [68], непонятно, являются ли они одорантами для этих ОР, и нет сведений о том, синтезируются ли подобные сигнальные молекулы, обладающие аналогичным запахом, в обонятельной слизи. То есть пока остается неясным, как это происходит на молекулярном уровне.
Полагают, чтобы управлять нацеливанием аксона ОСН, ОР взаимодействуют с несколькими молекулярными сигналами наведения. При этом ОР играют инструктивную роль в формировании сенсорной карты, в которой необходимо не только найти место для аксонов, экспрессирующих одинаковые ОР, но расположить эти гломерулы в определенном порядке в ОЛ. В этом процессе участвуют такие лиганды, как эфрины, особенно эфрин А3 и А5, и соответствующие им рецепторы Eph, а также рецептор Neuropilin1 (Nrp1) и его лиганд Semaphorine3A (Sema3A). Они экспрессируются на конусе роста аксонов ОСН и определяют гломерулярную локализацию вдоль переднезадней бульбарной оси [2, 66]. Сигналами для навигации ОСН вдоль дорсо-вентральной оси служат молекулы Robo-2-Slit-1. Robo-2, рецептор хеморепеллентов Slit, экспрессируется с высоким дорсомедиальным и низким вентролатеральным градиентом в ОЛ. Slit-1-Robo2 способствуют дорсальному нацеливанию ОСН. Slit-1 и Slit-3 необходимы для правильной иннервации вентральных бульбарных областей [2]. Neuropilin2 (Nrp2)-Semaphorine3F (Sema3F) также направляет аксоны к дорзальной области ОЛ [2]. Известно, что эти молекулы действуют как сигналы наведения аксонов для нескольких типов нейронов во время развития [69].
Необходимо отметить, что способность к пространственной сегрегации сенсорных афферентов вдоль переднезадней оси сильно коррелирует с экспрессией определенного типа ОР. ОСН, экспрессирующие один и тот же ОР, конвергируют с образованием гломерул на определенной стороне (медиальной или латеральной) каждой ОЛ. Таким образом, формируются две симметричные карты клубочков [2, 3]. Глобулярное расположение вдоль дорсо-вентральной оси ОЛ определяется местом ОСН в ОЭ. То есть ОСН, расположенные в самой дорсальной эпителиальной зоне, проецируют свои аксоны на самую дорсальную бульбарную часть, а ОСН в самой вентральной области ОЭ проецируют аксоны в вентральную область OЛ [48, 70, 71].
Таким образом, формирование обонятельной карты представляет собой многоэтапный процесс. Для этого процесса необходимы молекулы клеточной адгезии, экспрессия которых коррелирует с экспрессией ОР. Ими оказались молекулы адгезии Kirrel2/Kirrel3 и лиганды Ephrin-A и EphA. Они участвуют в конечном процессе привлечения аксонов, экспрессирующих один и тот же рецептор, для формирования индивидуальных гломерул [52].
Следует отметить, что в механизме наведения аксонов ОР играют инструктивную роль, дальнейшую функцию на себя берет цАМФ. Он активирует ПК-А, транслоцирующуюся в ядро для синтеза молекулярных рецепторов, реагирующих с молекулами наведения и молекулами адгезии. цАМФ в конусе роста, как уже отмечалось, синтезируется в результате активации ОР, расположенного в этой области ОСН. Доказано, что ОР в ОЖ и кончике аксона аналогичны. Однако пока неясно, если этим лигандом является тот же одорант, который активирует ОР на ОЖ, то как он оказывается в области конуса роста?
Область-мишень в ОЛ в значительной степени определяют факторы навигации, связанные с эпителиальной областью или “зоной”, в которой экспрессируется ОР. Если эти условия нарушаются на уровне рецепторного аппарата, это может привести к неправильному формированию нейронных связей в центральных отделах обонятельной сенсорной системы, а, следовательно, к нарушению обоняния. Возможно, этим можно объяснить ослабление обоняния вплоть до его отсутствия у людей, перенесших COVID-19. С одной стороны, у них преобладают незрелые рецепторные нейроны в ОЭ, а следовательно, меньше экспрессируется ОР. С другой стороны, у них может нарушаться синтез факторов наведения [72].
Как уже было сказано выше, ОР играют две разные роли в функционировании ОСН: обнаруживают пахучие молекулы и определяют выбор аксонов-мишеней в ОЛ. Таким образом, ОР не только преобразовывают химические раздражители из внешнего мира в электрические сигналы, но и определяют передачу этой информации в мозг, направляя формирование нейронной цепи в ОЛ посредством хемотаксиса кончика аксона в градиенте концентрации хемоаттрактанта. Следует отметить, что ОР как “хемосенсорный рецептор” функционирует также в сперме, где он модулирует движения жгутиков, влияющие на хемотаксис сперматозоидов [73, 74]. ОР участвует в хемотаксисе самих ОЖ, увеличивая вероятность взаимодействия с одорантом, а, следовательно, обонятельную чувствительность [11, 17, 63]. Эти данные свидетельствуют о том, что хемотаксические функции ОР сохраняются и, таким образом, используются несколькими системами для регуляции процессов, включающих подвижность и навигацию [75]. Однако процесс формирования обонятельной топографической карты эффективен, если имеются для этого важные условия – аминокислотная последовательность OР, уровень экспрессии рецепторного белка и время его экспрессии, которые являются важными детерминантами сортировки. Для конвергенции аксонов ОСН в гломерулах необходимо также сопряжение ОР с G-белком [34]. Нарушения сортировки аксонов в ОЛ, связанные с мутацией Golf, могут приводить к дисфункции обоняния. Вероятно, это может являться одной из причин ослабления обоняния при шизофрении, при которой мутирует Golf [76, 77].
Таким образом, уникальной особенностью организации обонятельной системы является двойная роль ОР: они улавливают запахи в эпителии носа и играют инструктивную роль в конвергенции аксонов ОСН в специфических локусах, т.е. гломерулах ОЛ.
Роль цАМФ
Факт того, что аксоны, принадлежащие обонятельным клеткам, несущим одинаковые ОР, конвергируют на специфические гломерулы в ОЛ и формируют таким образом топографическую сенсорную карту, установлен давно. Вместе с тем в настоящее время активно изучаются специфические молекулярные механизмы навигации аксонов к своим мишеням. Помимо роли, которую играют в этом процессе ОР, локализованные в аксонном холмике, внимание исследователей к хемотаксису аксонов привлекает участие цАМФ, синтезируемого в этой области ОСН.
Из вышеизложенного следует, что внутриклеточный сигнальный путь на конусе роста аксонов аналогичен таковому на ОЖ [11, 61, 63, 64, 68, 78].
Существуют убедительные доказательства центральной роли цАМФ в развитии сенсорной карты [33, 79, 80]. Методом генетической мутации с отменой синтеза цАМФ выявили, что аксоны, несущие эту мутацию, никогда не проникали в гломерулярный слой, а оставались в слое обонятельного нерва. Эта линия мышей была полностью лишена обоняния, и у них было изменено слияние сенсорных аксонов с образованием гломерул. Формирование и организация клубочков нарушались таким образом, что аксоны ОСН нацеливались на ОЛ, но не могли сформировать отчетливые и четко определенные гломерулы. Кроме того, многие клубочки были гетерогенными, поскольку содержали волокна, принадлежащие ОСН, экспрессирующим ОР к разным одорантам [66, 80, 81].
Это означает, что цАМФ, синтезируемый в кончике аксона ОСН под действием сигналов из ОЛ, регулирует экспрессию молекул его навигации к своей мишени в ОЛ [33, 81]. Одним из источников бульбарных сигналов, как полагают, являются ранние дифференцирующиеся проекционные нейроны, митральные клетки [82]. Каким образом цАМФ регулирует направленное движение аксона в градиенте концентрации хемоаттрактанта, т.e. хемотаксис аксона? Одним из предлагаемых специфических механизмов регуляции аксонального хемотаксиса является регуляция посредством изменения уровней цАМФ. Таким путем регулируются транскрипция и экспрессия таких молекул наведения аксонов, как Nrp1 и Sema3A, Kirrel2/3, эфрин-A/EphA [1, 78, 83]. При этом ОСН, экспрессирующие разные ОР, могут содержать разные уровни цАМФ. Именно от этого будет зависеть экспрессия тех молекул наведения, которые будут нацеливать аксоны в заданном направлении: по переднезадней или дорсо-вентральной оси ОЛ [84]. Процессы транскрипции осуществляются в ядре. Выше уже говорилось о том, что помимо локального действия цАМФ может функционировать на ядерном уровне. Активация аксональных ОР и повышение уровней цАМФ на кончике аксона активируют транслокацию ПК-А в ядро. Здесь этот ключевой фермент цАМФ-сигнального пути регулирует экспрессию генов молекул навигации аксона [11, 63].
Таким образом, цАМФ играет центральную роль в установлении нейронных связей в ОЛ. Если будут созданы соответствующие связи, нейрон продолжит свое созревание и выживет. Однако если соединения не установлены, возможно, из-за неправильного нацеливания, нейрон погибает под действием каспазы-8 [11].
Вместе с тем необходимо учитывать и то обстоятельство, что ОСН живут около 35 дней и погибают. Затем пул рецепторных клеток восстанавливается из базальных клеток. То есть ОЭ подвергается нейрогенезу на протяжении всей жизни [85, 86]. Поэтому для организации обонятельной топографической карты важен контроль за ее постоянным уточнением. Этот процесс регулируется сложным взаимодействием между активностью нейронов и молекулярными сигналами. Суть его заключается в том, что экспрессию рецепторов на поверхности растущих аксонов контролирует активность ОСН. Это позволяет аксонам дифференцированно изменять свои реакции на различные молекулы адгезии и наведения, экспрессируемые на аксонах, глии, внеклеточном матриксе и внутри ОЛ [84]. Нейронная активность, вызываемая стимуляцией одорантом, служит для непрерывного уточнения гломерулярной карты, тем самым предотвращая накопление ошибок навигации [87]. Однако вызванная запахом нейронная активность оказывает лишь ограниченное влияние на конвергенцию на клубочки, и, следовательно, на топографию ОЛ [11]. Она не оказывает существенного влияния на формирование сенсорной карты, что продемонстрировано в экспериментах на нескольких трансгенных линиях мышей, несущих генетические мутации таких ключевых элементов пути обонятельной трансдукции, как CNG-каналы [78, 88] или Golf [89]. При мутации в генах, кодирующих CNG-каналы и Golf, исчезают реакции на одоранты, но синтез цАМФ сохраняется, обеспечивая нормальное развитие сенсорной карты. Из этого следует, что если в ОСН сохраняется способность продуцировать цАМФ, то сохраняется возможность организации бульбарной топографической карты.
Оказалось, что важную роль в уточнении и поддержании топографической организации ОЛ играет спонтанная афферентная активность [63, 90–92]. Было установлено, что она играет важную роль не только в развитии, но и в поддержании топографии ОЛ и в обработке сенсорной информации [52, 90]. Нарушение спонтанной афферентной активности изменяет исходное нацеливание аксонов обонятельных клеток на ольфакторную луковицу [11].
Источником спонтанной активности служит наличие электропроводности в ОСН в “покое”. Известно, что мембраны ОСН земноводных в отсутствие пахучей стимуляции (“покой”) обладают электропроводностью порядка 158 пСм, которая обусловлена проницаемостью для ионов калия и кальция [93, 94]. В мембране ОСН обнаружены 4 типа K+-каналов с разной электропроводностью [95]. Однако от 20 до 40% электропроводности цилиарной мембраны связаны с токами, текущими через CNG-каналы, открытыми в “покое”. В изолированных нестимулируемых обонятельных клетках рыб, лягушек, крыс генерируются спонтанные потенциалы действия с частотой 100 в 1 мин. С ними связывают чувствительность к слабым стимулам [93]. Показано, что в “покое” ОЖ двигаются. У земноводных движения совершаются с участием механочувствительных, потенциал-зависимых и CNG-Са-каналов, открытых в отсутствие одорантов [17]. При этом спонтанная активация ОР в спонтанной активности ОСН не участвует. Эксперименты на специально созданной трансгенной линии мышей, экспрессирующих бета-адренергические рецепторы, доказали, что спонтанная активность ОСН поддерживается цАМФ [96].
Таким образом, в покоящихся ОСН, вероятно, благодаря двигательной активности ОЖ поддерживается определенный уровень цАМФ, участвующий в уточнении и поддержании топографической организации ОЛ [97].
Нейронные сети в ОЛ
В ОЛ образуются специфические связи, необходимые для нормальной работы мозга. В ней информация о запахе, закодированная в обонятельной карте, обрабатывается в локальных нейронных цепях, а затем передается митральными и пучковыми клетками в различные области обонятельной коры.
Развитие обонятельной карты регулируется сложным взаимодействием в пространстве и времени между молекулами навигации аксонов и активностью нейронов. Отличительной чертой зрелых гломерул (что представляет собой отличительную особенность в организации ОЛ) является то, что они иннервируются волокнами, экспрессирующими исключительно один и тот же ОР. Аксоны ОСН образуют синаптические соединения с несколькими мишенями в ОЛ, включая юкстагломерулярные, митральные и пучковые клетки, образуя внутригломерулярную сеть [1].
Различные типы клеток ОЛ образуют столбчатую структуру, при этом каждая гломерула вносит свой вклад в свою собственную колонку клеток. Поэтому “обонятельную колонку” определяют как функциональную единицу ОЛ. Эксперименты с сенсорной депривацией показали, что внутрибульбарная карта сохраняет высокий уровень пластичности на протяжении всей жизни. Аналогичный гломерулярной обонятельной карте уровень точности повторяется в карте второго порядка, состоящей из центральных нейронов [11].
Обонятельная кора интерпретирует карты запахов от ОЛ и переводит обонятельную информацию в поведенческие реакции. Каким образом? Синаптические входы от ОСН получают два типа проекционных нейронов в каждом клубочке: митральные и пучковые клетки. Поскольку каждая митральная/пучковая клетка посылает один первичный дендрит в специфическую гломерулу, каждая клетка специализируется для обработки обонятельных входов от одного вида ОР [57].
Синаптическая передача с аксонов ОСН на мишени в ОЛ осуществляется с участием цАМФ. Свои эффекты он проявляет посредством активации обонятельных пресинаптических CNG-каналов. Показано, что высокие концентрации цАМФ ингибируют синаптическую передачу с обонятельного нерва на эти мишени. Но циклонуклеотиды могут как подавлять, так и стимулировать синаптическую передачу посредством активации зависимых от них каналов. Поэтому CNG-каналы способны модулировать нейротрансмиссию. Следовательно, помимо регуляции сенсорной трансдукцией цАМФ может играть более широкую роль в ЦНС, нацеливая аксоны в нужном направлении и образуя интрабульбарные химические синапсы [46].
Вместе с тем методом электронно-микроскопической реконструкции было показано, что в ОЛ формируются электрические синапсы. Щелевые контакты образуются между дендритами митральных и юкстагломерулярных клеток и дендритами перигломерулярных клеток и между дендритами митральных и юкстагломерулярных клеток и дендритами других типов интернейронов [98]. Это означает, что в формирование нейронных сетей в ОЛ помимо химических включаются электрические синапсы, которые способствуют синхронности нейрональной активности в ОЛ, формируя локальные интрагломерулярные нейронные цепи [98–101]. И здесь цАМФ играет важную роль в установлении синаптических связей, а следовательно, в формировании нейронных цепей. Это связано с тем, что цАМФ регулирует механизм транспорта щелевых соединений для биогенеза функциональных электрических синапсов. На них приходится почти 20% связи в зрелой нервной системе [102].
Гломерула – функциональная единица для обработки обонятельной информации. Тонкая настройка уровней выходного сигнала от каждой гломерулы важна для процесса обработки ольфакторной информации в обонятельной сенсорной системе. Взаимодействие между нейрональными элементами в гломеруле может быть одним из основных механизмов, регулирующим этот уровень выходного сигнала [98]. Внутригломерулярные взаимодействия могут изменять силу сигнала в постсинаптических нейронах и изменять пространственный паттерн активности, вызванной запахом. Возбуждающие связи в клубочках в форме как щелевых, так и химических синаптических соединений могут приводить к усилению сигнала. Внутриклубочковое торможение может служить механизмом контроля усиления, действующим пресинаптически на синапсы ОСН или через постсинаптическое торможение основных клеток [1].
Если нарушается организация сенсорной карты в ОЛ, то могут изменяться связи между гомологичными гломерулами. В результате возможного неполного уточнения или неправильного нацеливания аксональных проекций внутрибульбарная связь может быть менее точной, а результирующая топографическая карта более размытой на ранних стадиях развития. Причиной неправильного формирования связей внутри ОЛ является ослабление спонтанной активности клеток. Как было сказано выше, нарушенная спонтанная активность влияет на уровни таких вторичных мессенджеров, как Ca2+ и циклические нуклеотиды, которые, как известно, играют критическую роль в нацеливании аксонов в ОЛ [90, 103]. Следовательно, можно предположить, что образование внутрибульбарных нейрональных цепей и организация анатомических и функциональных карт ОЛ будут зависеть от цАМФ. Их изменения будут влиять на обонятельное поведение [90]. Это означает, что дисфункция внутриклеточной сигнальной системы цАМФ в ОСН может влиять на обоняние.
Так, например, при шизофрении в обонятельном сенсорном эпителии обнаруживается значительное снижение экспрессии АЦ-3, а следовательно, сниженная продукция цАМФ. Неслучайно эти пациенты страдают пониженной обонятельной чувствительностью [104]. Кроме того, при некоторых психических заболеваниях затрудняется процесс распознавания запахов, свидетельствуя об ослаблении когнитивной функции [105]. Это связано с тем, что ОЭ тесно связан с важными лимбическими и нейроэндокринными областями головного мозга. Таким образом, механизмы обучения и памяти и механизмы обонятельного восприятия имеют общий анатомический субстрат. Цитоархитектурные аномалии в нем приводят к ослаблению обучения и памяти. Поэтому структурные нарушения в них будут сопровождаться обонятельной дисфункцией, а при ослаблении обоняния, в свою очередь, ухудшается познавательная деятельность, рабочая память, развивается депрессия, изменяются эмоции [106, 107].
В процессе запоминания, как известно, устанавливаются новые синаптические связи с образованием новых нейронных сетей. Синаптогенез не обходится без цАМФ. Его недостаток, по-видимому, формирует грубую связность сенсорной карты, отражающуюся на обонянии и способности к запоминанию информации [97, 102]. Нейронная сеть организована более сложно в центральном отделе обонятельного анализатора. Из ОЛ информация о запахе передается на обонятельную кору, в которую проецируют свои аксоны митральные и пучковые клетки. При этом информация о разных одорантах передается к определенным зонам коры, и в зависимости от области могут по-разному интерпретировать бульбарную карту запахов. То есть в пириформной коре выявили наличие пространственной организации, или кластеобразование, нейронов передней пириформной коры, возникающее от одного типа ОР у мышей. Следовательно, пространственное представительство одорантов в ОЛ может сохраняться в обонятельной коре [108]. Так, например, в обонятельном бугорке вместе с прилежащим вентральным полосатым телом формируется мотивационное поведение, связанное с запахом пищи и страхом. Выходные нейроны обонятельного бугорка проецируют свои аксоны на вентральный паллидум, нейроны которого, в свою очередь, посылают аксоны к дофаминергическим нейронам в вентральной области покрышки. Следовательно, информация о запахе пищи, обработанная в обонятельном бугорке, по-видимому, влияет на дофаминергические цепи ожидания вознаграждения [56]. Таким образом, целостность нейронных связей будет определять обонятельное поведение и, в конечном итоге, выживание вида. Пириформная кора сохраняет топографическую организацию пространственного кодирования запаха, которое возникает в гломерулах ОЛ.
Многие психические расстройства, к которым относится, например, шизофрения, связывают с нарушением синаптогенеза и пластичности [109]. В экспериментах на мышах с нокаутом показано, что при потере щелевых контактов развиваются дефекты электрических синапсов в мозжечке, вызывающие нарушения двигательной функции. Отсутствие электрических синапсов в гиппокампе нарушает процессы долгосрочной потенциации, сопровождаясь ослаблением обучения и памяти. Отсутствие щелевых контактов в коре приводит к десинхронизации корковых интернейронов и нарушению функции коры в зрительной и обонятельной сенсорных системах. Дисфункция электрических синапсов способствует этиологии таких расстройств, как аутизм и эпилепсия [110]. Следовательно, можно предположить, что в их основе лежит нарушение функции внутриклеточной системы цАМФ в нейронах. Подтверждением этого служит анализ многочисленных клинических и экспериментальных данных, который привел исследователей к выводу, что патогенетические механизмы развития шизофрении связаны с нарушением регуляции цАМФ [111].
ЗАКЛЮЧЕНИЕ
Обнаружение пахучих сигналов из окружающей среды и формирование соответствующих поведенческих реакций в ответ на эти сигналы характеризуют функцию обонятельной сенсорной системы. ОСН, расположенные в полости носа, проецируют аксоны, которые синаптически соединяются с дендритами нейронов второго порядка в ОЛ, которые, в свою очередь, передают собранную информацию в области мозга более высокого порядка. Образующиеся соединения между рецепторными клетками и ОЛ удивительно точны. Аксоны ОСН, экспрессирующие один и тот же ОР, иннервируют специфические клубочки внутри сложной трехмерной структуры ОЛ, локализующихся в определенных координатах в ее дорсо-вентральной, переднезадней и медиолатеральной осях.
Таким образом, связи между ОСН и специфическими для данного ОР гломерулами, образующие “обонятельные колонки”, межглобулярные связи и связи между ОЛ и обонятельными центрами более высокого порядка образуют нейронные сети, в формировании которых участвует цАМФ. Их нарушение в результате разрегулирования системы цАМФ вызывает нарушение функции нейронов ЦНС с последующим дефицитом когнитивной функции при психических заболеваниях.
Список литературы
Murthy, Venkatesh N (2011) Olfactory maps in the brain. Annu Rev Neurosci 34: 233–258. https://doi.org/10.1146/annurev-neuro-061010-113738
Francia S, Lodovichi C (2021) The role of the odorant receptors in the formation of the sensory map. BMC Biol 19 (1): 174. https://doi.org/10.1186/s12915-021-01116-y
Carr WES (1988) The Molecular Nature of Chemical Stimuli in the Aquatic Environment. In: Atema, J, Fay RR, Popper AN, Tavolga WN (eds) Sensory Biology of Aquatic Animals. Springer. New York. https://doi.org/10.1007/978-1-4612-3714-3_1
Бронштейн АА (1977) Обонятельные рецепторы позвоночных. Л. Наука. [Bronshtein AA (1977) Olfactory receptors in vertebrates. L. Nauka. (In Russ)].
Cunningham AM, Manis PB, Reed RR, Ronnet GV (1999) Olfactory receptor neurons exist as distinct subclasses of immature and mature cells in primary culture. Neurosci 93: 1301–1312.
Buck L, Axel R (1991) A novel multigene family may encode odorant receptors: a molecular basis for odor recognition. Cell 65: 175–187.
Zhang R, Wang P, Yu S, Hansbro P, Wang H (2020) Computerized screening of G-protein coupled receptors to identify and characterize olfactory receptors. J Toxicol Environment Health Part A 83: 1–11. https://doi.org/10.1080/15287394.2019.1709305
Yu Y, Ma Z, PJ, Xu L, Li W, Belloir C, Topin J, Briand L, Golebiowski J, Cong X (2022) Extracellular loop 2 of G protein–coupled olfactory receptors is critical for odorant recognition. J Biol Chem 298: 1–10. https://doi.org/10.1016/j.jbc.2022.102331
Kurian SM, Naressi RG, Manoel D, Barwich AS, Malnic B, Saraiva LR (2021) Odor coding in the mammalian olfactory epithelium. Cell Tissue Res 383 (1): 445–456. https://doi.org/10.1007/s00441-020-03327-1
Ikegami K, de March CA, Nagai MH, Ghosh S, Do M, Sharm R, Bruguera ES, Lu YE, Fukutania Y, Vaidehid N, Yohd M, Matsunami H (2020) Structural instability and divergence from conserved residues underlie intracellular retention of mammalian odorant receptors. PNAS 117: 2957–2967. https://doi.org/10.1073/pnas.1915520117
Lodovichi C, Belluscio L (2012) Odorant Receptors in the Formation of the Olfactory Bulb Circuitry. Physiology 27: 200–212. https://doi.org/10.1152/physiol.00015.2012
Omura M, Takabatake Y, Lempert E, Benjamin-Hong S, D`Hulst C, Feinstein P (2022) A genetic platform for functionally profiling odorant receptors in olfactory cilia ex vivo. Science Signal 15: eabm6112. https://doi.org/10.1126/scisignal.abm6112
Hayden S, Teeling EC (2014) The Molecular Biology of Vertebrate Olfaction. Anat Record 297: 2216–2226. https://doi.org/10.1002/ar.23031
Kim B-R, Rha M-S, Cho H-J, Yoon J-H, Kim C-H (2023) Spatiotemporal dynamics of the development of mouse olfactory system from prenatal to postnatal period. Front Neuroanat 17: 1157224. https://doi.org/10.3389/fnana.2023.1157224
Barry WA, Janet M (2005) Young Olfaction: Diverse Species, Conserved Principles Review. Neuron 48: 417–430. https://doi.org/10.1016/j.neuron.2005.10.022
Connelly T, Yua Y, Grosmaitre X, Wang J, Santarelli LC, Savigner A, Qiao X, Wang Z, Storm DR, Ma M (2017) G protein-coupled odorant receptors underlie mechanosensitivity in mammalian olfactory sensory neurons. Proc Natl Acad Sci U S A 112: 590–595. https://doi.org/10.1073/pnas.1418515112
Bigdaj EV, Fufachev DK, Petrov PR, Samojlov VO (2017) Mechanisms of Electromechanical and Electrochemical Coupling in Olfactory Cilia of the Frog (Rana temporaria). Biophysics 62: 240–246. https://doi.org/10.1134/S0006350917020051
Liu Q, Li S, Lu C, Yu CR, Huang L (2018) G protein γ subunit Gγ13 is essential for olfactory function and aggressive behavior in mice. Neuroreport 29: 1333–1339. https://doi.org/10.1097/WNR.0000000000001122
Li R-C, Molday LL, Lin C-C, Yau K-W (2022) Low signaling efficiency from receptor to effector in olfactory transduction: A quantified ligand-triggered GPCR pathway. Proc Natl Acad Sci U S A 119: e2121225119. https://doi.org/10.1073/pnas.2121225119
Genovese F, Reisert J, Kefalov VJ (2021) Sensory Transduction in Photoreceptors and Olfactory Sensory Neurons: Common Features and Distinct Characteristics. Front Cell Neurosci 15: 761416. https://doi.org/10.3389/fncel.2021.761416
Cong X, Ren W, Pacalon J, Xu R, Xu L, Li X, de March CA, Matsunami H, Yu H, Yu Y, Golebiowsk J (2022) Large-Scale G Protein-Coupled Olfactory Receptor–Ligand Pairing. ACS Cent Sci 8: 379–387. https://doi.org/10.1021/acscentsci.1c01495
Jimenez RC, Casajuana-Martin N, García-Recio A, Alcántara L, Pardo L, Campillo M, Gonzalez A (2021) The mutational landscape of human olfactory G protein-coupled receptors. BMC Biology 19: 21. https://doi.org/10.1186/s12915-021-00962-0
Li F, Ponissery-Saidu S, Yee KK, Wang H, Chen M-L, Iguchi N, Zhang G, Jiang P, Reisert J, Huang L (2013) Heterotrimeric G Protein Subunit G 13 Is Critical to Olfaction. J Neurosci 33: 7975–7984. https://doi.org/10.1523/JNEUROSCI.5563-12.2013
Wong ST, Trinh K, Hacker B, Chan GCK, Lowe G, Gaggar A, Xia Z, Gold GH, Storm DR (2000) Disruption of the Type III Adenylyl Cyclase Gene Leads to Peripheral and Behavioral Anosmia in Transgenic Mice. Neuron 27: 487–497. https://doi.org/10.1016/s0896-6273(00)00060-x
Ostrom KF, LaVigne JE, Brust TF, Seifert R, Dessauer CW, Watts VJ, Ostrom RS (2022) Physiological roles of mammalian transmembrane adenylyl cyclase isoforms. Physiol Rev 102 (2): 815–857. https://doi.org/10.1152/physrev.00013.2021
Hanoune J, Defer N (2001) Regulation and role of adenylyl cyclase isoforms. Annu Rev Pharmacol Toxicol 41: 145–174. https://doi.org/10.1146/annurev.pharmtox.41.1.145
Bakalyar HA, Reed RR (1990) Identification of a specialized adenylyl cyclase that may mediate odorant detection. Science 250: 1403–1406. https://doi.org/10.1126/science.2255909
Devasani K, Yao Y (2022) Expression and functions of adenylyl cyclases in the CNS. Fluids Barriers CNS 19: 23. https://doi.org/10.1186/s12987-022-00322-2
Liu X, Zhou Y, Li S, Yang D, Jiao M, Liu X, Wang Z (2020) Type 3 adenylyl cyclase in the main olfactory epithelium participates in depression-like and anxiety-like behaviours. J Affect Disord 268: 28–38. https://doi.org/10.1016/j.jad.2020.02.041
Qiu L, LeBel RP, Storm DR, Chen X (2016) Type 3 adenylyl cyclase: a key enzyme mediating the cAMP signaling in neuronal cilia. Int J Physiol Pathophysiol Pharmacol 8: 95–108.
Challis RC, Tian H, Yin W, Ma M (2016) Genetic Ablation of Type III Adenylyl Cyclase Exerts Region-Specific Effects on Cilia Architecture in the Mouse Nose. PLoS One 11: e0150638. https://doi.org/10.1371/journal.pone.0150638
Ou Y, Ruan Y, Cheng M, Moser JJ, Rattner JB, van der Hoorn FA (2009) Adenylate cyclase regulates elongation of mammalian primary cilia. Exp Cell Res 315: 2802–2817. https://doi.org/10.1016/j.yexcr.2009.06.028
Chesler AT, Zou D-J, Le Pichon CE, Peterlin ZA, Matthews GA, Pei X, Miller MC, Firestein S (2007) A G protein/cAMP signal cascade is required for axonal convergence into olfactory glomeruli. Proc Natl Acad Sci U S A 104: 1039–1044. https://doi.org/10.1073/pnas.0609215104
Kulaga HM, Leitch CC, Eichers ER, Badano JL, Lesemann A, Hoskins BE, Lupski JR, Beales PL, Reed RR, Katsanis N (2004) Loss of BBS proteins causes anosmia in humans and defects in olfactory cilia structure and function in the mouse. Nat Genet 36: 994–998. https://doi.org/10.1038/ng1418
Mair RG, Gestel RC, Blank DL (1982) Changes in morphology and physiology of olfactory receptor cilia during development. Neuroscience 7: 3091–3103. https://doi.org/10.1016/0306-4522(82)90232-9
Cao G, Lam H, Jude JA, Karmacharya N, Kan M, Jester W, Koziol-White C, Himes BE, Chupp GL, An SS, Panettieri RA Jr (2022) Inhibition of ABCC1 Decreases cAMP Egress and Promotes Human Airway Smooth Muscle Cell Relaxation. Am J Respir Cell Mol Biol 66(1): 96–106. https://doi.org/10.1165/rcmb.2021-0345OC
Erofeeva N, Meshalkina D, Firsov M (2023) Multiple Roles of cAMP in Vertebrate Retina. Cells 12 (8): 1157. https://doi.org/10.3390/cells12081157
Patra, C, Foster K, Corley JE, Dimri M, Brady MF (2022) Biochemistry, cAMP. In: StatPearls. StatPearls Publ.
Slika H, Mansour H, Nasser SA, Shaito A, Kobeissy F, Orekhov AN, Pintus G, Eid AH (2023). Epac as a tractable therapeutic target. Eur J Pharmacol 945: 175645. https://doi.org/10.1016/j.ejphar.2023.175645
Boczek, T, Cameron EG, Yu W, Xia X, Shah SH, Castillo Chabeco B, Galvao J, Nahmou M, Li J, Thakur H, Goldberg JL, Kapiloff MS (2019) Regulation of Neuronal Survival and Axon Growth by a Perinuclear cAMP Compartment. J Neurosci 39 (28): 5466–5480. https://doi.org/10.1523/JNEUROSCI.2752-18.2019
Pun RYK, Kleene SJ (2003) Contribution of Cyclic-Nucleotide-Gated Channels to the Resting Conductance of Olfactory Receptor Neurons. Biophys J 84: 3425–3435. https://doi.org/10.1016/S0006-3495(03)70064-2
Zaccolo M, Di Benedetto G, Lissandron V, Mancuso L, Terrin A, Zamparo I (2006) Restricted diffusion of a freely diffusible second messenger: mechanisms underlying compartmentalized cAMP signaling. Biochem Soc Trans 34: 495–497. https://doi.org/10.1042/BST0340495
Di Benedetto G, Iannucci LF, Surdo NC, Zanin S, Conca F, Grisan F, Gerbino A, Lefkimmiatis K (2021) Compartmentalized Signaling in Aging and Neurodegeneration. Cells 10 (2): 464. https://doi.org/10.3390/cells10020464
Turetsky BI, Moberg PJ (2009) An odor-specific threshold deficit implicates abnormal intracellular cyclic AMP signaling in schizophrenia. Am J Psychiatry 166: 226–233. https://doi.org/10.1176/appi.ajp.2008.07071210
Ronnett GV, Moon C (2002) G proteins and olfactory signal transduction. Annu Rev Physiol 64: 189–222. https://doi.org/10.1146/annurev.physiol.64.082701.102219
Murphy GJ, Isaacson JS (2003) Presynaptic Cyclic Nucleotide-Gated Ion Channels Modulate Neurotransmission in the Mammalian Olfactory Bulb. Neuron 37: 639–647. https://doi.org/10.1016/s0896-6273(03)00057-6
Takeuchi H, Kurahashi T (2005) Mechanism of Signal Amplification in the Olfactory Sensory Cilia. J Neurosci 25: 11084–11091. https://doi.org/10.1523/JNEUROSCI.1931-05.2005
Ressler KJ, Sullivan SL, Buck LB (1994) A molecular dissection of spatial patterning in the olfactory system. Curr Opin Neurobiol 4: 588–596. https://doi.org/10.1016/0959-4388(94)90061-2
Takeuchi H, Kurahashi T (2003) Identification of second messenger mediating signal transduction in the olfactory receptor cells. J Gen Physiol 122: 557–567. https://doi.org/10.1085/jgp.200308911
Breer H (1993) Olfactory receptor cells: recognition and transduction of chemical signals. Cytotechnology 11: 13–16. https://doi.org/10.1007/BF00749052
Serizawa S, Miyamichi K, Nakatani H, Suzuki M, Saito M, Yoshihara Y, Sakano H (2003) Negative feedback regulation ensures the one receptor-one olfactory neuron rule in mouse. Science 302: 2088–2094. https://doi.org/10.1126/science.1089122
Serizawa S, Miyamichi K, Takeuchi H, Yamagishi Y, Suzuki M, Sakano H (2006) A Neuronal Identity Code for the Odorant Receptor-Specific and Activity-Dependent Axon Sorting. Cell 127: 1057–1069. https://doi.org/10.1016/j.cell.2006.10.031
Mombaerts P (1996) Targeting olfaction. Curr Opin Neurobiol 6: 481–486. https://doi.org/10.1016/s0959-4388(96)80053-5
Munger SD, Leinders-Zufall T, Zufal F (2009) Subsystem Organization of the Mammalian Sense of Smell. Annu Rev Physiol 71: 115–140. https://doi.org/10.1146/annurev.physiol.70.113006.100608
Rodriguez-Gil DJ, Bartel DL, Jaspers AW, Mobley AS, Imamura F, Greer CA (2015) Odorant receptors regulate the final glomerular coalescence of olfactory sensory neuron axons. Proc Natl Acad Sci U S A 112: 5821–5826. https://doi.org/10.1073/pnas.1417955112
Mori K, Sakano H (2011) How Is the Olfactory Map Formed and Interpreted in the Mammalian Brain? Annu Rev Neurosci 34: 467–499. https://doi.org/10.1146/annurev-neuro-112210-112917
Firestein S (2001) How the olfactory system makes sense of scents. Nature 413: 211–218. https://doi.org/10.1038/35093026
Belluscio L, Lodovichi C, Feinstein P, Mombaerts P, Katz LC (2002) Odorant receptors instruct functional circuitry in the mouse olfactory bulb. Nature 419: 296–300. https:// doi.org/https://doi.org/10.1038/nature01001
Wachowiak M, Cohen LB (2001) Representation of Odorants by Receptor Neuron Input to the Mouse Olfactory Bulb. Neuron 32: 723–735. https://doi.org/10.1002/1096-9861(20001009)426:1<68::aid-cne5>3.0.co;2-z10.1002/1096-9861(20001009)426:1<68::aid-cne5>3.0.co;2-zhttps://doi.org/10.1016/s0896-6273(01)00506-2
Treloar HB, Feinstein P, Mombaerts P, Greer CA (2002) Specificity of glomerular targeting by olfactory sensory axons. J Neurosci 22: 2469–2477. https://doi.org/10.1523/JNEUROSCI.22-07-02469.2002
Wang F, Nemes A, Mendelsohn M, Axel R (1998) Odorant receptors govern the formation of a precise topographic map. Cell 93: 47–60. https://doi.org/10.1016/s0092-8674(00)81145-9
Strotmann J, Levai O, Fleischer J, Schwarzenbacher K, Breer H (2004) Olfactory Receptor Proteins in Axonal Processes of Chemosensory Neurons. J Neurosci 24: 7754–7761. https://doi.org/10.1523/JNEUROSCI.2588-04.2004
Lodovichi C (2020) Role of Axonal Odorant Receptors in Olfactory Topography. Neurosci Insights 15: 2633105520923411. https://doi.org/10.1177/2633105520923411
Maritan M, Monaco G, Zamparo I, Zaccolo M, Pozzan T, Lodovichi C (2009) Odorant receptors at the growth cone are coupled to localized cAMP and Ca2+ increases. Proc Natl Acad Sci U S A 106: 3537–3542. https://doi.org/10.1073/pnas.0813224106
Greer PL, Greenberg ME (2008) From synapse to nucleus: calcium-dependent gene transcription in the control of synapse development and function. Neuron 59: 846–860. https://doi.org/10.1016/j.neuron.2008.09.002
Imai T, Suzuki M, Sakano H (2006) Odorant receptor-derived cAMP signals direct axonal targeting. Science 314: 657–661. https://doi.org/10.1126/science.1131794
Dang P, Fisher SA, Stefanik DJ, Kim J, Raper JA (2018) Coordination of olfactory receptor choice with guidance receptor expression and function in olfactory sensory neurons. PLoS Genet 14: e1007164. https://doi.org/10.1371/journal.pgen.1007164
Zamparo I, Francia S, Franchi SA, Redolfi N, Costanzi E, Kerstens A, Fukutani Y, Battistutta R, Polverino de Laureto P, Munck S, De Strooper B, Matsunami H, Lodovichi C (2019) Axonal odorant receptors mediate axon targeting. Cell Rep 29: 4334–4348. https://doi.org/10.1016/j.celrep.2019.11.099
Takeuchi H, Sakano H (2014) Neural map formation in the mouse olfactory system. Cell Mol Life Sci 71: 3049–3057. https://doi.org/10.1007/s00018-014-1597-0
Astic L, Saucier D (1986) Anatomical mapping of the neuroepithelial projection to the olfactory bulb in the rat. Brain Res Bull 16: 445–454. https://doi.org/10.1016/0361-9230(86)90172-3
Tsuboi A, Miyazaki T, Imai T, Sakano H (2006) Olfactory sensory neurons expressing class I odorant receptors converge their axons on an antero-dorsal domain of the olfactory bulb in the mouse. Eur J Neurosci 23: 1436–1444. https://doi.org/10.1111/j.1460-9568.2006.04675.x
Бигдай ЕВ, Самойлов ВО (2020) Обонятельная дисфункция как индикатор ранней стадии заболевания COVID-19. Интеграт физиол 1: 187–195. [Bigdai EV, Samoilov VO (2020) Olfactory dysfunction as an early indicator of COVID-19 disease. Integrat fiziol 1: 187–195. (In Russ)]. https://doi.org/10.33910/2687-1270-2020-1-3-187-195
Kaupp UB, Alvarez L (2016) Sperm as microswimmers – navigation and sensing at the physical limit. Eur Phys J Spec Top 225: 2119–2139. https://doi.org/10.1140/epjst/e2016-60097-1
Ali MA, Wang Y, Qin Z, Yuan X, Zhang Y, Zeng C (2021) Odorant and Taste Receptors in Sperm Chemotaxis and Cryopreservation: Roles and Implications in Sperm Capacitation, Motility and Fertility. Genes 12 (4): 488. https://doi.org/10.3390/genes12040488
Oh SJ (2018) System-Wide Expression and Function of Olfactory Receptors in Mammals. Genomics Inform 16: 2–9. https://doi.org/10.5808/GI.2018.16.1.2
Borgmann-Winter KE, Wang H-Y, Ray R, Willis BR, Moberg PJ, Rawson NE, Gur RE, Turetsky BI, Hahn C-G (2016) Altered G Protein Coupling in Olfactory Neuroepithelial Cells From Patients With Schizophrenia. Schizophr Bull 42: 377–385. https://doi.org/10.1093/schbul/sbv129
Бигдай ЕВ, Самойлов ВО, Синегубов АА (2021) Комплексные нарушения обонятельной сенсорной системы при шизофрении. Успехи физиол наук 52: 93–104. [Bigdai EV, Samoilov VO, Sinegubov AA (2021) Complex disorders of the olfactory sensory system in schizophrenia. Uspekhi fiziol nauk 52: 93–104. (In Russ)]. https://doi.org/10.31857/S0301179821020028
Zheng C, Feinstein P, Bozza T, Rodriguez I, Mombaerts P (2000) Peripheral olfactory projections are differentially affected in mice deficient in a cyclic nucleotide-gated channel subunit. Neuron 26: 81–91. https://doi.org/10.1016/S0896-6273(00)81140-X
Sakano H (2020) Developmental regulation of olfactory circuit formation in mice. Dev Growth Differ 62: 199–213. https://doi.org/10.1111/dgd.12657
Col JAD, Matsuo T, Storm DR, Rodriguez I (2007) Adenylyl cyclase-dependent axonal targeting in the olfactory system. Development 134: 2481–2489. https://doi.org/10.1242/dev.006346
Zou D-J, Chesler AT, Le Pichon CE, Kuznetsov A, Pei X, Hwang EL, Firestein S (2007) Absence of adenylyl cyclase 3 perturbs peripheral olfactory projections in mice. J Neurosci 27: 6675–6683. https://doi.org/10.1523/JNEUROSCI.0699-07.2007
Key B, ST John J (2002) Axon Navigation in the Mammalian Primary Olfactory Pathway: Where to Next. Chem Senses 27: 245–260. https://doi.org/10.1093/chemse/27.3.245
Nakashima A, Ihara N, Shigeta M, Kiyonari H, Ikegaya Y, Takeuchi H (2019) Structured spike series specify gene expression patterns for olfactory circuit formation. Science 365: eaaw5030. https://doi.org/10.1126/science.aaw5030
Cho JH, Prince JEA, Cloutier J-F (2009) Axon guidance events in the wiring of the mammalian olfactory system. Mol Neurobiol 39: 1–9. https://doi.org/10.1007/s12035-008-8047-7
van der Linden CJ, Gupta P, Bhuiya AI, Riddick KR, Hossain K, Santoro SW (2020) Olfactory Stimulation Regulates the Birth of Neurons That Express Specific Odorant Receptors. Cell Rep 33: 1–26. https://doi.org/10.1016/j.celrep.2020.108210
Durante MA, Kurtenbach S, Sargi ZB, Harbour JW, Choi R, Kurtenbach S, Goss GM, Matsunami H, Goldstein BJ (2020) Single-cell analysis of olfactory neurogenesis and differentiation in adult humans. Nature Neurosci 23 (3): 323–326. https://doi.org/10.1038/s41593-020-0587-9
Dang, P, Fisher SA, Stefanik DJ, Kim J, Raper JA (2018) Coordination of olfactory receptor choice with guidance receptor expression and function in olfactory sensory neurons. PLoS Genetics 14 (1): e1007164. https://doi.org/10.1371/journal.pgen.1007164
Feinstein P, Bozza T, Rodriguez I, Vassalli A, Mombaerts P (2004) Axon guidance of mouse olfactory sensory neurons by odorant receptors and the beta2-adrenergic receptor. Cell 117: 833–846. https://doi.org/10.1016/j.cell.2004.05.013
Belluscio L, Gold GH, Nemes A, Axel R (1998) Mice deficient in G(olf) are anosmic. Neuron 20: 69–81. https://doi.org/10.1016/S0896-6273(00)80435-3
Lorenzon P, Redolfi N, Podolsky MJ, Zamparo I, Franchi SA, Pietra G, Boccaccio A, Menin A, Murthy VN, Lodovichi C (2015) Circuit formation and function in the olfactory bulb of mice with reduced spontaneous afferent activity. J Neurosci 35: 146–160. https://doi.org/10.1523/JNEUROSCI.0613-14.2015
Redolfi N, Lodovichi C (2021) Spontaneous afferent activity carves olfactory circuits. Front Cell Neurosci 15: 637536. https://doi.org/10.3389/fncel.2.021.637536
Qiu Q, Wu Y, Ma L, Xu W, Hills M Jr, Ramalingam V, Yu CR (2021) Acquisition of innate odor preference depends on spontaneous and experiential activities during critical period. eLife 10: e60546. https://doi.org/10.7554/eLife.60546
Kleene SJ (2008) The electrochemical basis of odor transduction in vertebrate olfactory cilia. Chem Senses 33: 839–859. https://doi.org/10.1093/chemse/bjn048
Lindemann B (2001) Predicted profiles of ion concentrations in olfactory cilia in the steady state. Biophys J 80: 1712–1721. https://doi.org/10.1016/S0006-3495(01)76142-5
Miyamoto T, Restrepo D, Teeter J (1992) Voltage-dependent and odorant-regulated currents in isolated olfactory receptor neurons of the channel catfish. J Gen Physiol 99: 505–529. https://doi.org/10.1085/jgp.99.4.505
Nakashima A, Takeuchi H, Imai T, Saito H, Kiyonari H, Abe T, Chen M, Weinstein LS, Yu CR, Storm DR, Nishizumi H, Sakano H (2013) Agonist-independent GPCR activity regulates anterior-posterior targeting of olfactory sensory neurons. Cell 154: 1314–1325. https://doi.org/10.1016/j.cell.2013.08.033
Song HJ, Ming GL, Poo MM (1997) cAMP-induced switching in turning direction of nerve growth cones. Nature 388: 275–279. https://doi.org/10.1038/40864
Kosaka T, Kosaka K (2005) Intraglomerular dendritic link connected by gap junctions and chemical synapses in the mouse main olfactory bulb: Electron microscopic serial section analyses. Neuroscience 131: 611–625. https://doi.org/10.1016/j.neuroscience.2004
Vaughn MJ, Haas JS (2022) On the Diverse Functions of Electrical Synapses. Front Cell Neurosci 16: 910015. https://doi.org/10.3389/fncel.2022.910015
Schubert C, Schulz K, Träger S, Plath A-L, Omriouate A, Rosenkranz SC, Morellini F, Friese MA, Hirnet D (2022) Neuronal Adenosine A1 Receptor is Critical for Olfactory Function but Unable to Attenuate Olfactory Dysfunction in Neuroinflammation. Front Cell Neurosci 16: 912030. https://doi.org/10.3389/fncel.2022.912030
Tavakoli A, Schmaltz A, Schwarz D, Margrie TW, Schaefer AT, Kollo M (2018) Quantitative Association of Anatomical and Functional Classes of Olfactory Bulb Neurons. J Neurosci 38(33): 7204–7220. https://doi.org/10.1523/JNEUROSCI.0303-18.2018
Palumbos SD, Skelton R, McWhirter R, Mitchel A, Swann I, Von Stetin SHS, Miller DM (2021) cAMP controls a trafficking mechanism that maintains the neuron specificity and subcellular placement of electrical synapses. Development Cell 56: 3235–3249. https://doi.org/10.1016/j.devcel.2021.10.011
West AE, Greenberg ME (2011) Neuronal activity-regulated gene transcription in synapse development and cognitive function. Cold Spring Harb Perspect Biol 3: a005744. https://doi.org/10.1101/cshperspect.a005744
Chen X, Xu J, Li B, Guo W, Zhang J, Hu J (2018) Olfactory impairment in first-episode schizophrenia: a case-control study, and sex dimorphism in the relationship between olfactory impairment and psychotic symptoms. BMC Psychiatry 18 (1): 199. https://doi.org/10.1186/s12888-018-1786-8
Dahmani L, Patel RM, Yang Y, Chakravarty MM, Fellows LK, Bohbot VD (2018) An intrinsic association between olfactory identification and spatial memory in humans. Nat Commun 9: 4162. https://doi.org/10.1038/s41467-018-06569-4
Zald DH, Pardo JV (1997) Emotion, olfaction, and the human amygdala: amygdala activation during aversive olfactory stimulation. Proc Natl Acad Sci U S A 94: 4119–4124. https://doi.org/10.1073/pnas.94.8.4119
Le Coz M, Lévêque L, Da Silva MP, Le Callet P (2022) From Olfaction to Emotions: An Interactive and Immersive Experience. In: ACM IMX Workshop EmotionIMX: Considering Emotions in Multimedia Experience – ACM Int Confer on Interact Media Exp: IMX. 127–133. https://doi.org/10.6084/m9.figshare.20069384.v1
Zelano C, Sobel N (2005) Humans as an animal model review for systems-level organization of olfaction. Neuron 48: 431–454. https://doi.org/10.1016/j.neuron.2005.10.009
Obi-Nagata K, Temma Y, Hayashi-Takagi A (2019) Synaptic functions and their disruption in schizophrenia: From clinical evidence to synaptic optogenetics in an animal model. Proc Jpn Acad Ser B Phys Biol Sci 95: 179–197. https://doi.org/10.2183/pjab.95.014
Martin E, Lasseigne AM, Miller AC (2020) Understanding the Molecular and Cell Biological Mechanisms of Electrical Synapse Formation. Front Neuroanat 14: 12. https://doi.org/10.3389/fnana.2020.00012
Tardito D, Tura GB, Bocchio L, Bignotti S, Pioli R, Racagni G, Perez J (2000) Abnormal levels of cAMP-dependent protein kinase regulatory subunits in platelets from schizophrenic patients. Neuropsychopharmacology 23: 216–219. https://doi.org/10.1016/S0893-133X(99)00161-X
Дополнительные материалы отсутствуют.
Инструменты
Журнал эволюционной биохимии и физиологии