Физиология растений, 2023, T. 70, № 6, стр. 563-576
Пора неспецифической проницаемости (mPTP) в митохондриях растений и ее роль в гибели клеток
П. А. Буцанец a, *, Н. А. Шугаева a, А. Г. Шугаев a, **
a Федеральное государственное бюджетное учреждение науки Институт физиологии растений
им. К.А. Тимирязева Российской академии наук
Москва, Россия
* E-mail: p.corbeau@list.ru
** E-mail: ag_shugaev@ifr.moscow
Поступила в редакцию 01.05.2023
После доработки 29.05.2023
Принята к публикации 29.05.2023
- EDN: FEPPOD
- DOI: 10.31857/S0015330323600341
Аннотация
В обзоре изложены современные представления о строении, механизмах регуляции и функциональной роли поры неспецифической проницаемости (mPTP) во внутренней мембране митохондрий животных и растений. Приведены некоторые особенности, характеризующие функционирование mPTP в митохондриях растений и ее регуляцию под влиянием Са2+ и АФК. Суммированы имеющиеся в литературе доказательства, свидетельствующие об участии митохондрий в программируемой гибели клеток растений, в том числе благодаря индукции mPTP. Намечены направления дальнейших исследований mPTP в митохондриях растений.
ВВЕДЕНИЕ
Митохондрии являются центральными органеллами дыхания и энергетики клетки. Осуществляя превращение органических соединений и синтез АТФ, необходимый для поддержания жизнедеятельности клетки, митохондрии, вместе с тем, в неблагоприятных условиях, способны активировать ее запрограммированную гибель (PCD) [1]. Кроме того, митохондриям отводится основная роль во внутриклеточном сигналинге, в котором участвуют вторичные мессенджеры, например, Ca2+ или АФК [2, 3]. Важно отметить, что внутренняя митохондриальная мембрана (IMM) в соответствии с хемиосмотической концепцией Митчелла непроницаема не только для протонов, но практически для всех метаболитов и сигнальных молекул, а для их трансмембранного транспорта требуются специальные переносчики или каналы. При этом внешняя митохондриальная мембрана (ОММ) более проницаема для ионов и растворенных веществ, что опосредованно наличием потенциал-зависимого анионного канала (VDAC) и других селективных каналов [4–6].
В ранних работах было показано, что при определенных условиях проницаемость IMM может резко возрастать, тогда протоны и различные ионы, а также небольшие молекулы могут свободно проходить в матрикс органелл. Это явление (изменение проницаемости внутренней мембраны) получило название митохондриальной пермеабилизации (mPT). Существование mPT в митохондриях животных впервые было обнаружено и изучено Haworth и Hunter [7–9]. Вначале это открытие было воспринято как артефакт, связанный с повреждением митохондрий, однако позднее было доказано, что mPT возникает в результате открытия во внутренней мембране специального мегаканала или поры (mPTP) под действием различных факторов (например, при накоплении Ca2+ в матриксе) [10]. Благодаря постоянному интересу исследователей, за более чем 40-летнюю историю изучения этого канала было опубликовано несколько тысяч статей. Оказалось, что нарушение функционирования mPTP приводит к дисфункции митохондрий, вследствие чего возникают многие болезни человека: от болезни Альцгеймера и ишемической болезни сердца, до онкологических и нейродегенеративных заболеваний [11, 12]. Поэтому результаты изучения этого мегаканала важны не только для фундаментальной науки, но также для лучшего понимания природы многих патологий, включая, по-видимому, болезни растений (см. ниже). Поскольку наибольшего прогресса в изучении структуры, механизмов регуляции и функциональной роли mPTP удалось достичь на митохондриях животных, представляется необходимым вначале дать ее краткую характеристику в этих органеллах.
СТРОЕНИЕ, РЕГУЛЯЦИЯ И ФУНКЦИЯ mРТР В МИТОХОНДРИЯХ ЖИВОТНЫХ
У животных mPTP определяют, как Са2+-зависимое и циклоспорин А-чувствительное увеличение проницаемости внутренней митохондриальной мембраны для протонов и веществ с молекулярной массой меньше 1.5 кДа различной природы: низкомолекулярных ионов, сахаров, нуклеотидов и т.д. [11, 12]. Состояние mPTP регулируется различными модуляторами, которые либо ингибируют (циклоспорин A, АДФ, АТФ, НАД·Н, Mg2+, H+ и др.) или активируют (Са2+, жирные кислоты, Pi, АФК и др.) ее открытие [10–14]. Учитывая важную роль этого канала, механизмы, регулирующие его переход из закрытого в открытое состояние, чрезвычайно сложны и не до конца изучены. В разных обзорах приводится более 40 различных эффекторов, способных оказывать прямое или опосредованное влияние на активность mPTP в митохондриях животных [10–13].
Наиболее важным регуляторным компонентом mPTP является шаперон циклофилин Д [10, 12, 13, 15]. В присутствии Pi этот белок связывается с субъединицей АТФ-синтазы OSCP, что приводит к открытию mPTP. Циклоспорин A (ЦсA), путем связывания с циклофилином Д индуцирует его отсоединение от АТФ-синтазы, блокируя образование поры [13, 15]. Чувствительность к ЦсА часто служит диагностическим тестом функционирования поры в митохондриях животных, однако в митохондриях растений такое ингибирование наблюдается не всегда (см. ниже).
Ключевую роль в регуляции mРТР в митохондриях животных играют ионы Ca2+. Повышение концентрации Ca2+ в матриксе, наряду с увеличением уровня АФК, приводит к открытию поры, коллапсу mΔΨ и набуханию органелл [10, 12, 14, 16–18]. Хорошо известно, что Ca2+, являясь активатором открытия mРТР в физиологических условиях, способен накапливаться в митохондриях в большом количестве без увеличения проводимости внутренней мембраны. Pазличные эндогенные модуляторы mРТP способны изменять порог концентрации Ca2+, необходимой для инициации данного процесса. Адениновые нуклеотиды (АДФ, АТФ и АМФ), вероятно, являются наиболее мощными эндогенными ингибиторами mРТ, увеличивая порог концентрации Ca2+, при котором происходит спонтанное открытие поры [7–10, 18]. В отличие от Ca2+ другие двухвалентные катионы Sr2+, Mn2+ и Ba2+ являются сильными ингибиторами mPT, препятствуя притоку Ca2+ внутрь митохондрий, а Mg2+ тормозит открытие поры путем конкурентного ингибирования [7–10]. Недавно было показано, что Ca2+ связывается c АТФ-синтазой на участке близко расположенном к сайту связывания Mg2+ между α- и β-субъединицами [14, 18].
Другими важными эндогенными регуляторами открытия mPTP являются АФК, а также активные формы азота (АФА). Известно, что митохондрии являются, как основным источником, так и мишенью для АФК/АФА. Показано, что mРТР чрезвычайно чувствительна к изменению своего редокс-состояния. Окислительный стресс, наиболее изученный индуктор mPTP. АФК и прооксиданты способствуют Ca2+-индуцированному открытию mPTP, вызывая изменение редокс-состояния SH-групп в молекулах белков, формирующих или регулирующих активность mРТР во внутренней мембране митохондрий животных [1, 17–19]. Обнаружена высокая чувствительность к АФК F1F0-АТФ-синтазе, являющейся, в соответствии с современными представлениями, основным компонентом mРТР в митохондриях животных [20, 21].
Кроме того, индукция mРТР регулируется величиной mΔΨ и ∆рН матрикса, конформацией транслокатора адениновых нуклеотидов (АNТ) и другими эффекторами [10]. Как и адениновые нуклеотиды, высокий mΔΨ удерживает поры закрытыми, в то время как его снижение способствует спонтанной индукции mPT. Подобный контроль mРТР со стороны электрохимического градиента протонов (∆µH+) создает концептуальную основу для “примирения” или согласования существования mPTP с хемиосмотической теорией, главным постулатом которой, как известно, является непроницаемость внутренней мембраны митохондрий для протонов [12]. Показано, что ингибиторы ANT − атрактилозид и бонгкрековая кислота противоположным образом модулируют функционирование mPTP, соответственно, способствуя и подавляя ее открытие. Эти результаты послужили одной из предпосылок для предположения об участии ANT в формировании mPTP [10, 12].
Уже в ранних работах было показано, что у животных mPTP может функционировать в двух основных состояниях: с низкой (с амплитудой 0.3–0.7 nS) и высокой (1.5 nS) проводимостью [22, 23]. Открытие mРТР в состоянии высокой проводимости или “классической” mPTP (p-mPTP), носит долговременный и, как правило, необратимый характер. Оно сопровождается полной диссипацией mΔΨ на внутренней мембране, нарушением ионного и осмотического гомеостаза в матриксе, высокоамплитудным набуханием митохондрий, нарушением целостности внешней мембраны органелл и высвобождением из межмембранного пространства ряда проапоптозных белков (цитохрома с, белка AIF и других). Эти белки могут запускать процесс апоптоза посредством активации соответствующих протеаз (каспаз) и нуклеаз в цитозоле [1, 12, 16, 22, 24]. В состоянии высокой проводимости mPTP проявляется, как правило, в стрессовых ситуациях, когда наблюдается серьезное нарушение работы митохондрий, связанное, например, с повышенной генераций органеллами АФК и возникновением окислительного стресса [1, 18, 20]. Если это происходит с отдельными митохондриями, коллапс mΔΨ может активировать митофагию, целью которой является удаление поврежденных органелл (так называемый “механизм контроля качества митохондрий”) [12]. В том случае, когда индукция p-mРТР охватывает большую часть популяции митохондрий, которое сопровождается увеличением объема матрикса, исчезновением крист, а также пермеабилизацией ОММ вследствие активации ОММ-порообразующих белков (ВАХ, ВАК), запускается процесс PCD по пути апоптоза или некроза в зависимости от количества оставшихся в клетке неповрежденных митохондрий, способных поддерживать синтез АТФ [12, 18].
В состоянии низкой проводимости индукция mPTP увеличивает проницаемость IMM для протонов и, по-видимому, небольших катионов (К+ Na+, Ca2+), что ведет к ее частичной или полной деполяризации, а также разобщению окислительного фосфорилирования. Показано, что отличительными особенностями этого подсостояния mРТР (или t-mPTP) являются: легкая обратимость, нечувствительность к экзогенному Са2+, слабая чувствительность к ЦсА и отсутствие набухания митохондрий [21, 23, 25, 26]. Открытие mРТР может быть как кратко-, так и долговременным [23, 24]. При этом именно долговременное открытие mРТР в любой из двух конформаций ведет к необратимым функциональным изменениям в митохондриях и фактически определяет судьбу клетки [22, 23, 27]. Индукция mPTP в состоянии низкой проводимости возникает спонтанно в процессе нормальной физиологической активности митохондрий (например, в результате накопления Ca2+ внутри органелл). В результате поступления Н+ из цитозоля в митохондрии снижается pH матрикса, что способствует закрытию поры. В таких условиях mPTP может быстро переходить из закрытого состояния в открытое и обратно в результате перераспределения ионов между цитозолем и матриксом митохондрий [18, 25, 28]. Быстрый циклический переход из открытого в закрытое состояние t-mPTP, которое называют “мерцанием” (flickering) поры, можно регистрировать с использованием флуориметричеcкой техники в изолированных митохондриях и интактных клетках [23, 28, 29]. Вопрос о том, может ли открытие t-mРТР катализировать пассивный выход ионов Ca2+ из матрикса в цитозоль по градиенту его концентрации остается дискуссионным. Тем не менее, в ряде работ показано, что в состоянии низкой проводимости mРТР проницаема не только для протонов, но и для низкомолекулярных катионов (К+, Na+, и, по-видимому, Са2+). В этом состоянии функционирование mРТР может участвовать в передаче Са2+-сигнала и/или в регуляции (гомеостатировании) уровня Са2+ в матриксе за счет его быстрого выхода из органелл [12, 22, 30]. Таким образом, кратковременную индукцию поры в состоянии t-mPTP, по-видимому, можно рассматривать в качестве протекторного механизма, предохраняющего митохондрии от избыточного накопления Ca2+ в матриксе и от Ca2+-зависимых изменений метаболизма органелл, например, повышенного образования АФК [18].
Несмотря на значительный прогресс, достигнутый в изучении факторов, регулирующих индукцию mPTP, открытым остается вопрос о ее структуре. Согласно более ранним представлениям, структурными компонентами mPTP, формируемой в месте контакта митохондриальных мембран, выступали вольт-зависимый анионный канал (VDAC) или порин (внешняя мембрана), а также АNТ (внутренняя мембрана) и ассоциированный со стороны матрикса консервативный белок циклофилин Д [18, 31, 32]. Позднее было высказано предположение, что в структуру mPTP входит переносчик фосфата, который, связываясь с циклофилином Д и ANT, образует во внутренней мембране митохондрий пору, регулируемую ионами Ca2+ [10, 27]. Однако указанные модели строения mPTP не нашли своего подтверждения в ходе генетических исследований. Было показано, что функционирование mPTP сохранялось в митохондриях, выделенных из трансгенных растений, у которых экспрессия гена (или нескольких генов), кодирующих каждый из указанных выше структурных компонентов была подавлена [18, 33, 34].
Более современные представления о структуре mPTP связаны с предположением о формировании mPTP с участием F0F1-AТФ-синтазы [12, 13, 18, 26]. В настоящее время предлагаются две рабочие модели высокоамплитудной поры: “гипотеза димера” и “гипотеза с-кольца”. Согласно первой, Ca2+-индуцированное изменение конформации димера вызывает образование поры на границе двух мономеров АТФ-синтазы [35]. Вторая гипотеза предполагает формирование канала в результате конформационных изменений в кольце с-субъединиц ATФ-синтазы, индуцированных ионами Ca2+ [36]. Имеется несколько прямых доказательств того, что АТФ-синтаза участвует в образовании mPTP. Во-первых, изолированная АТФ-синтаза или ее с-субьединица (или ее димер), встроенные в искусственный бислой, генерируют Ca2+-индуцируемый поток веществ высокой проводимости, который электрофизиологически эквивалентен индукции p-mPTP [35, 36]. Кроме того, генетические изменения субъединиц АТФ-синтазы заметно влияют на чувствительность mPTP к ее регуляторам [36–39].
Недавно, с использованием генетических методов были получены доказательства того, что ANT и АТФ-синтаза могут быть независимыми каналами, ответственными за функционирование mPTP в состоянии низкой и высокой проводимости, соответственно [40–42]. Основываясь на этих данных, была предложена новая интересная модель строения mРТР, которая синтетически соединила две другие модели, ранее привлекшие наибольшее внимание исследователей. Согласно новой модели структура mPTP формируется из двух основных функциональных элементов, ANT и ATФ-синтазы, объединенных в единый комплекс под названием ATФ-синтасома (ATP synthasome) (рис. 1) [13, 18]. Можно не сомневаться, что эта новейшая модель строения mPTP также привлечет большое внимание митохондриологов и будет подвергнута всестороннему изучению и проверке.
Рис. 1.
Гипотетическое строение поры неспецифической проницаемости (mPTP) во внутренней мембране митохондрий в виде АTФ-синтасомы − комплекса, состоящего из димера АТФ-синтазы (ATPase) и аденилатного транспортера (ANT). При функционировании комплекса в состоянии высокой проводимости (p-mPTP) канал, проницаемый для растворов веществ с молекулярной массой меньше 1.5 кДа, образуется, или между двумя молекулами ATФ–синтазы, или внутри ее мономера, по-видимому, в кольце c-субъединиц (толстые стрелки). В состоянии низкой проводимости (t-mPTP), канал, проницаемый только для протонов и, возможно, небольших катионов, включая Са2+, формируется внутри переносчика аденилатов (тонкая стрелка).
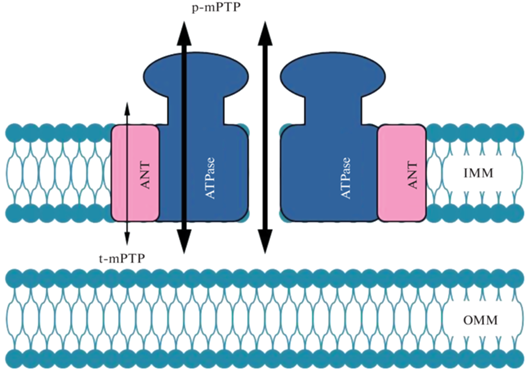
ОСОБЕННОСТИ ФУНКЦИОНИРОВАНИЯ mРТР В МИТОХОНДРИЯХ РАСТЕНИЙ
За более чем 25 лет прошедших после первого сообщения о присутствии mРТР в митохондриях растений (этиолированных стеблей гороха) [43], данные о присутствии и свойствах этой поры были получены при изучении митохондрий, выделенных из: листьев овса [44], клубней картофеля [45, 46], корней пшеницы [47], клеточной культуры Arabidopsis thaliana [48], листьев канабиса [49], семядолей люпина [50] и некоторых других растительных объектов. Судя по этим данным, mРТР в митохондриях растений, как и в митохондриях животных, может функционировать в двух метаболических состояниях (или подсостояниях) – высокой и низкой проводимости. Например, функционирование индуцируемой Са2+ и Pi типичной (или канонической) mРТР было показано в митохондриях клубней картофеля, корней пшеницы, листьев овса и некоторых других растений [44, 45, 47]. Открытие mРТР в этих органеллах сопровождалось коллапсом m∆Ψ, а также высокоамплитудным набуханием матрикса с последующим выходом из органелл цитохрома с. При этом, индукция mРТР, например, в митохондриях клубней картофеля ингибировалась ЦсА который, как уже отмечалось, служит ключевым тестом для идентификации активности mРТР в митохондриях животных [46]. Интересно, что в митохондриях клубней картофеля и корней пшеницы Са2+-зависимая индукция mРТР и выход из митохондрий цитохрома с наблюдались в условиях недостатка кислорода в среде инкубации [46, 47]. При этом сама по себе аноксия была не способна индуцировать открытие mРТР и потерю митохондриями цитохрома с [47]. Кроме того, Са2+-индуцируемое набухание митохондрий тормозилось при снижении рН (с 7.4 до 6.6) и активировалось в присутствии прооксидантов (диамида и фениларсен-оксида), а также линоленовой кислоты, что в целом аналогично условиям, способствующим индукции mРТР в митохондриях животных [46]. Вместе с тем, сразу же обнаружились и некоторые особенности, характеризующие mРТР в митохондриях растений.
Во-первых, для Са2+-зависимой индукции mРТР было необходимо присутствие в среде инкубации высокой концентрации Са2+ (0.5–2.5 мМ), что, по мнению авторов, могло объясняться низкой активностью переносчика этого иона (Са2+-унипортера) в митохондриях, например, клубней картофеля, корней пшеницы и листьев овса [45–47]. В других случаях Са2+-зависимая индукция mРТР достигалась благодаря присутствию Са2+-ионофоров, например, А23187, обеспечивающего транспорт и накопление Са2+ в матриксе митохондрий листьев овса [44]. Во-вторых, чувствительность mРТР к ЦсА в митохондриях, выделенных, например, из клубней картофеля проявлялась в присутствии мощного восстановителя – ДТТ [46]. В другой работе, индукция mРТР в состоянии высокой проводимости (р-mРТР) в митохондриях корней пшеницы оказалась не чувствительной к ЦсА [47]. Причина различной чувствительности mРТР в растительных митохондриях к ЦсА остается неизвестной, хотя белки семейства циклофилина Д, а также фермент пептидилпролил цис-транс изомераза (peptidylprolyl cis-trans isomerase), через взаимодействие с которыми реализуется регуляторное действие ЦсА на активность mРТР в митохондриях животных, обнаружены и в растениях, например, в митохондриях Arabidopsis thaliana и Glycine max [45, 51, 52]. В недавней работе сотрудников лаборатории A. Vianello [53] также было показано, что регуляция mРТР под влиянием ЦсА осуществлялась в результате его прямого взаимодействия с циклофилином Д в митохондриях стеблей гороха.
Особенностью функционирования mРТР в митохондриях стеблей гороха, которая индуцировалась после накопления в матриксе (с помощью Са2+ ионофора ETH129) 240-720 мкМ экзогенного Са2+ и сопровождалась диссипацией m∆Ψ, является то, что она в отличие, например, от митохондрий клубней картофеля не сопровождалась набуханием матрикса и потерей цитохрома с. Это характерно для функционирования поры в состоянии низкой проводимости [53]. Кроме того, было показано, что кроме ионов Cа2+, активаторами mРТР, которые повышали ее чувствительность (“sensitized”) к основным регуляторам в этом состоянии, являлись прооксиданты, например, диамид и фениларсен-оксид, а кроме ЦсА индукция mРТР ингибировалась в присутствии субстратов АТФ-синтазы (Mg-AДФ и Mg-AТФ). По мнению авторов это означает, что механизмы, регулирующие изменение проницаемости внутренней мембраны в митохондриях гороха в результате индукции mPTP в состоянии низкой проводимости (t-mPTP), близки к таковым, модулирующим активность типичной mРТР в состоянии высокой проводимости (p-mPTP) в митохондриях животных [53]. Нами были изучены условия индукции и некоторые свойства mРТР, функционирующей в состоянии низкой проводимости в митохондриях семядолей люпина [50, 54]. Важно, что эти митохондрии отвечали основным критериям нативности, в частности, характеризовались интактными мембранами, а также прочным сопряжением процессов окисления и фосфорилирования. При окислении дыхательных субстратов ЦТК, в частности, сукцината, митохондрии семядолей были способны длительное время поддерживать m∆Ψ, как за счет работы ЭТЦ, так и в ходе АТФ-азной реакции при ингибировании дыхательной цепи, например, в условиях гипоксии. Показано, что добавление в инкубационную среду дышащих митохондрий люпина салициловой кислоты (СК), активирующей образование АФК, или прооксиданта (фениларсен-оксида), или 50–100 мкМ CaCl2, вызывало после непродолжительного лаг-периода (8–10 мин), полную диссипацию m∆Ψ, указывая на индукцию протонной проводимости внутренней мембраны, которая не сопровождалась набуханием органелл [50, 54]. Также было обнаружено, что коллапс m∆Ψ под действием Cа2+ и прооксидантов наблюдался при торможении ЭТЦ в условиях анаэробиоза и полностью обращался при аэрации среды инкубации. Кроме того, диссипация m∆Ψ не ингибировалась в присутствии ЦсА, но полностью предотвращалась благодаря присутствию в среде инкубации ДТТ или хелаторов Са2+ (ЭГТА и ЭДТА). На основании полученных результатов авторы пришли к заключению, что в митохондриях семядолей люпина индукция mРТР в состоянии низкой проводимости происходила при повышении в матриксе уровня АФК и Са2+, а также при торможении работы ЭТЦ в условиях гипоксии, которые, по-видимому, дополнительно повышают чувствительность (“сенсибилизируют”) поры к ее активаторам, в частности, к ионам Cа2+ [54]. Следует также отметить, что митохондрии семядолей люпина могут служить удобной моделью для изучения свойств mРТР, поскольку способны без использования Са2+ ионофоров поглощать и накапливать экзогенный Cа2+, хотя и с более низкой скоростью, чем митохондрии животных (печени крысы). Это важно, так как одной из главных трудностей в активации mРТР в митохондриях, выделенных из многих растительных объектов, является, как уже отмечалось, низкая активность механизмов, обеспечивающих транспорт в матрикс экзогенного Са2+, в первую очередь, низкая активность в этих органеллах Са2+-унипортера [46, 53].
Несмотря на полное отсутствие работ, специально посвященных изучению молекулярной структуры РТ поры в митохондриях растений, общепринято, что она не должна существенно отличаться от таковой в митохондриях животных и дрожжей, учитывая сходство основных механизмов регуляции их активности [25, 55]. Кроме того, в митохондриях растений присутствуют все те же основные компоненты mРТР, которые в разное время были включены в ее структуру: VDAC, транслокатор адениновых нуклеотидов (ANT), циклофилин Д, переносчик Pi, АТФ-синтаза и др. [55]. В этой связи следует отметить еще одно обстоятельство. Как отмечено выше, в митохондриях животных и растений функционирование mРТP в различном состоянии регулируется примерно одинаковым способом, т.е. активируется Са2+, АФК, прооксидантами, тиол-окисляющими агентами, аноксией, а тормозится в присутствии ЦсА (хотя у растений не всегда), антиоксидантов, ДТТ, аденилатов, а также при снижении рН среды инкубации органелл. Учитывая, что активность mРТР в состоянии высокой и низкой проводимости, согласно современным представлениям, катализируют два различных канала, образуемых соответственно АТФ-синтазой и ANT, подобное сходство в регуляции их активности может свидетельствовать о близком контакте указанных переносчиков в интактных митохондриях растений. Действительно, как уже было отмечено выше, имеются данные о том, что в митохондриях животных АTФ-синтаза образует стабильную связь или близкий контакт с ANT и переносчиком фосфата, формируя, таким образом, ATФ-синтасому [18]. Полагают, что благодаря такому строению mРТР возможна, например, механическая передача регуляторного воздействия Са2+ через соответствующие Са2+-связывающие центры АТФ-синтазы и изменение ее конформации, на изменение конформации ANT, катализирующего при наличии дополнительных условий функционирование поры в состоянии низкой проводимости [18]. Не исключено присутствие AТФ-синтасомы и в митохондриях растений, поскольку механизмы регуляции ее функционирования в состоянии низкой и высокой проводимости также имеют много общего.
Вызывает интерес участие некоторых стрессовых фитогормонов, прежде всего, СК и фитомелатонина в качестве возможных регуляторов mPTP у растений, благодаря, прежде всего, их влиянию на образование АФК органеллами и поддержание редокс-гомеостаза в различных компартментах клетки, включая митохондрии. Показано, что при накоплении эндогенной СК в клетках растений в условиях биотического и абиотического стресса, а также при действии экзогенной СК на изолированные митохондрии, она активирует образование АФК, в частности, перекиси водорода, которая является вторичным посредником передачи сигнала фитогормона и стимулирует экспрессию генов антиоксидантных ферментов и других защитных белков [56–59].
Однако если антиоксидантная защита клетки не срабатывает (например, при дальнейшем накоплении СК), в митохондриях возникает окислительный стресс. В этом случае СК способна ингибировать ЭТЦ и синтез АТФ, что ведет к дальнейшему повышению уровня АФК в митохондриях и клетке с последующей индукцией PCD [56, 60, 61]. Кроме того, было показано, что СК может индуцировать АФК-зависимую пермеабилизацию внутренней мембраны митохондрий животных и растений вследствие открытия в ней mPTP, функционирование которой приводит к полной деполяризации внутренней мембраны, а в митохондриях печени крысы также к высокоамплитудному набуханию органелл и выходу из матрикса цитохрома с [50, 62]. Возможен и другой путь индукции пермеабилизации внутренней мембраны под влиянием СК, не связанный с генерацией АФК. В работе Belt с соавт. [58] показано, что СК взаимодействует с ЭТЦ митохондрий растений на уровне убихинона, который является одним из регуляторов активности mРTР в митохондриях животных [12].
Фитомелатонин, присутствие которого у растений было показано немногим более 25 лет назад, будучи мощным антиоксидантом, прямо и косвенно вовлечен в процессы редокс-регуляции в митохондриях и клетках, особенно в стрессовых условиях [63, 64]. Многочисленные исследования показали, что мелатонин во взаимодействии с другими фитогормонами, играет ключевую роль в повышении иммунитета растений и формировании неспецифической устойчивости к действию различных видов абиотического и биотического стресса [63, 65]. Однако механизмы, посредством которых этот индоламин повышает стресс-устойчивость растений, остаются не до конца выясненными. Вместе с тем, весьма вероятно, что, как и в случае с СК, мишенью (или одной из мишеней) действия мелатонина станут митохондрии, которые, наряду с хлоропластами, являются местом биосинтеза этого гормона. Показано, например, что обработка мелатонином (200 нМ) предотвращала накопление АФК, ДНК фрагментацию, выход цитохрома с из митохондрий и Pb-индуцируемую PCD в клеточной культуре табака линии BY 2 [66]. Нами было показано, что мелатонин способен существенно ингибировать образование АФК (перекиси водорода) митохондриями семядолей люпина при окислении сукцината [59]. Интересно, что обработка проростков A. thaliana мелатонином помимо индукции экспрессии генов антиоксидантных белков (аскорбатпероксидазы и каталазы), также активировала процесс аутофагии. Это рассматривается авторами как дополнительный способ защиты растений мелатонином, способствующий их выживанию в условиях окислительного стресса, когда антиоксидантная система не срабатывает и необходимо удаление из клетки поврежденных белков [67].
На митохондриях животных (скелетных мышц) показано, что мелатонин (10-4–10-6 М) предотвращал индуцируемую прооксидантами деполяризацию митохондрий и открытие р-mРТР, путем снижения ее чувствительности к ионам Са2+ [68]. На митохондриях мозга крысы обнаружено, что мелатонин и первичные продукты его метаболизации, в частности циклический 3-гидроксимелатонин, не ингибировали открытие mРТР, а стабилизировали ее в состоянии низкой проводимости (t-mPTP), что, по мнению авторов, может играть важную протекторную роль в условиях окислительного стресса, предотвращая избыточное накопление в матриксе Cа2+, открытие mРТР в состоянии высокой проводимости и индукцию апоптоза [69]. К сожалению, в литературе пока практически нет данных о влиянии фитомелатонина на функционирование mРТР в митохондриях растений.
ВОЗМОЖНОЕ УЧАСТИЕ МИТОХОНДРИЙ И ИНДУКЦИИ mРТР В ПРОЦЕССЕ ПРОГРАММИРУЕМОЙ СМЕРТИ КЛЕТОК РАСТЕНИЙ (PCD)
Если присутствие mРТР в митохондриях растений не вызывает сомнений, выяснение ее функциональной роли, например, в процессе PCD у этих организмов, в настоящее время, сопровождается оживленными дискуссиями. Известно, что у животных и растений функционируют различные пути, ведущие к смерти клеток, как в процессе нормального развития организма, так и в результате действия на него различных стрессовых факторов. По аналогии с основными типами PCD у животных (апоптоз, некроз и аутофагия), выделяют три пути (или типа) PCD у растений: апоптозо-подобный (AL-PCD), некрозо-подобный (NL-PCD) или не лизосомный PCD, и аутофагию (Autophagy) [70, 71]. Постулируется, что у растений отсутствует ряд отличительных морфологических признаков, характеризующих апоптоз в клетках животных, таких как формирование апоптозных телец и их поглощение (engulfment) в ходе фагоцитоза лизосомами соседних клеток [70]. Кроме того, у растений отсутствуют некоторые биохимические механизмы, обеспечивающие индукцию и прохождение “классического” апоптоза с участием митохондрий животных. В частности, у них не были найдены гены белков семейства Bcl-2, регулирующих проницаемость внешней мембраны митохондрий животных, а также гены специфических протеаз − каспаз, активируемых проапоптозными белками (цит. с и АIF), выходящими из митохондрий при пермеабилизации внешней мембраны [70, 72]. Поэтому, в классическом варианте этого понятия апоптоз у растений пока не обнаружен, а процесс AL-PCD по некоторым параметрам более близок к mРТР-индуцируемому некроптозу у животных, разновидности mPCD, в которой важную роль играет повышение в матриксе уровня Cа2+, а также генерация АФК митохондриями и пермеабилизация внутренней мембраны органелл в результате открытия ЦсА-чувствительной mРТP [73]. Тем не менее, термин AL-PCD традиционно используются применительно к растениям, подчеркивая, в том числе, консервативность начальных этапов этого типа PCD, проходящих с участием митохондрий (образование пор в мембранах органелл, диссипация m∆Ψ, выход в цитозоль цитохрома с и активация протеаз), которые были обнаружены в клетках животных, растений и дрожжей. Кстати, по мнению некоторых авторов, у растений нет и типичной аутофагии, если строго придерживаться определения этого процесса PCD для животных [70].
В последнее время высказываются сомнения относительно корректности использования термина AL-PCD для обозначения одного из путей PCD у растений. Например, исходя из отсутствия влияния ЦсА и ЭГТА на индукцию mPCD высокой температурой в протопластах клеток табака линии BY-2, а также следя за изменениями их морфологии, высказывается мнение, что у растений апоптозный путь смерти клеток не сохранился, а основным способом их утилизации является процесс аутофагии, т.е. “самопереваривания” клеточного содержимого, протекающий внутри вакуолей [74]. Несомненно, приведенные выше факты говорят о том, что процесс апоптоза в клетках растений существенно модифицирован. Однако представление о том, что в растениях полностью отсутствует AL-PCD, включая его начальную стадию, в которой ключевую регуляторную роль играют митохондрии, на наш взгляд, недостаточно обосновано. Во-первых, в растениях обнаружены апоптозо-подобные тельца, кроме того, появляются доказательства, что вакуоль может быть ключевым звеном на пути удаления поврежденных растительных клеток и клеточных структур, т.е. выполнять функции, аналогичные фагоцитозу у животных в ходе апоптоза [75]. Во-вторых, это представление противоречит большому количеству других экспериментальных данных, полученных при изучении процесса mPCD, идущего по пути AL-PCD во многих растительных объектах и индуцируемого различными стресс-факторами [75].
Следует сразу отметить, что, в отличие от животных, роль митохондрий растений в процессах PCD остается недостаточно изученной. Тем не менее, этому вопросу, уделялось и уделяется довольно много внимания. Наиболее часто в таких публикациях, которые суммированы в нижеприведенных обзорах, находила подтверждение важная или даже определяющая роль митохондрий, включая индукцию mРТР, в процессе гибели клеток растений, идущему по пути AL-PCD [75–80]. В указанных обзорах приведены и проанализированы характерные морфологические и биохимические признаки AL-PCD в клетках растений, которые аналогичны процессу апоптоза: уменьшение (конденсация) объема клетки (протопласта), при сохранении целостности плазмалеммы, конденсация хроматина с последующей олигомеризацией молекул ДНК вследствие активации эндонуклеаз и появление “ДНК-лестницы” (DNA-laddering) в гелях при электрофорезе, повышенная генерация АФК митохондриями, индукция p-РТР и диссипация m∆Ψ, нарушение целостности мембран, выход в цитозоль цитохрома с, AIF и других белков, локализованных в межмембранном пространстве, а также активация каспазо-подобных протеаз (метакаспаз). Важно, что во многих случаях была продемонстрирована чувствительность процесса PCD к ЦсА и другим известным ингибиторам mPTP.
Во многих исследованиях на различных модельных растительных объектах и клеточных культурах в условиях in vivo были продемонстрированы диссипация m∆Ψ, выход цитохрома с, AIF, ДНК нуклеазы из межмембранного пространства митохондрий, с последующей фрагментацией ядерной ДНК и индукцией гибели клеток в ходе развития растений (формирование ксилемы и трахеид) [81], при действии на растения: абиотического стресса (высокой температуры, засоления, ультрафиолета) [80–84], различных метаболитов (маннозы, протопорфирина IX) [85, 86], фитогормонов (например, СК) [87], а также вирусных токсинов и индукторов реакции сверхчувствительности (викторина, гарпина) [2, 88]. Кроме того, о роли mPTP в увеличении проницаемости мембран митохондрий и индукции PCD у растений свидетельствовало предотвращение или замедление по времени начала диссипации m∆Ψ, выхода цитохрома с и набухания митохондрий после обработки растений ее ингибитором − ЦсА [44, 48, 83–85, 87, 89]. В частности, было показано, что избыток ультрафиолета индуцировал PCD в протопластах A. thaliana [84]. В этом процессе участвовали каспазо-подобные протеазы, он сопровождался конденсацией и фрагментацией ядер, межнуклеосомной деградацией ДНК, появлением “ДНК-лестницы” и диссипацией m∆Ψ митохондрий. С использованием методов микроскопии и флуоресцентных индикаторов было обнаружено, что обработка растений UV-C индуцировала резкую активацию образования АФК митохондриями и хлоропластами. А прединкубация растений c аскорбиновой кислотой и ЦсA существенно замедляла развитие PCD и снижала процент протопластов подверженных гибели после облучения UV-C [84]. В другой работе, с использованием проточной цитометрии было изучено участие митохондрий в индукции PCD в протопластах табака при действии солевого стресса. Показано, что обработка протопластов 200 мМ NaCl приводила к резкому увеличению образования АФК митохондриями и диссипации m∆Ψ, которые регистрировались до появления признаков PCD (“ДНК-лестницы”), и которые ингибировались предобработкой растений ЦсА [83].
Имеются многочисленные данные о важной роли митохондрий в защите растений от болезней, вызванных патогенами, вирусными токсинами и элиситорами [88–91]. Биотический стресс, вызванный атакой биотрофных патогенов или их токсинов индуцирует резкую ответную реакцию организма – реакцию сверхчувствительности (СВЧ), которая сопровождается созданием в пораженной ткани (например, листа) локальной защитной зоны, состоящей из мертвых клеток. Судя по имеющейся литературе можно заключить, что в ходе СВЧ митохондрии играют ключевую роль в регуляции PCD, суммируя и интегрируя многие сигналы, поступающие в клетку различными путями [89, 90]. Среди таких сигналов могут быть, стресс-индуцируемое повышение в цитозоле уровня Са2+, а также АФК, NO· и других АФА, накопление стрессовых фитогормонов, например, СК, действие которых на митохондрии определяет дальнейшую судьбу клетки. Неоднократно было показано, что в зоне поражения ткани гибель клеток проходит также по пути AL-PCD, поскольку она сопровождается конденсацией ядер, фрагментацией ДНК, потерей m∆Ψ митохондрий, выходом из них цитохрома с и активацией каспазо-подобных протеаз, которая частично обращается обработкой растений ЦсА [44, 75, 88–90]. Учитывая, что в литературе до недавнего времени отсутствовали данные о способности цитохрома с или других белков, выходящих из митохондрий, активировать протеазы в цитозоле растительных клеток, высказывалось мнение, что СВЧ-индуцируемый выход цитохрома с из митохондрий может отражать конечную стадию дисфункции органелл, быть причиной повышенной генерации ими АФК, но не являться непосредственным триггером PCD [90].
Однако не исключено, что положение дел с возможностью прямого взаимодействия (и активации?) цитохрома с и протеаз или других белков, участвующих в процессе PCD у растений, может серьезно измениться. Во-первых, было показано, что, хотя канонические каспазы отсутствуют у растений, тем не менее, протеазы, действительно, вовлечены в процесс PCD у растений, демонстрируя при этом каспазо-подобную специфичность [92, 93]. Во-вторых, с использованием методов протеомики было обнаружено, что цитохром с A. thaliana в условиях in vitro может специфически контактировать в цитозоле растительных и животных клеток с десятью различными белками, участвующими в различных биохимических процессах (белкового фолдинга, регуляции трансляции и т.д.), в том числе, с протеазами, играющими ключевую роль в процессе PCD [94]. Наконец, недавно была обнаружена еще одна интересная мишень цитохрома с – это изоформа белка 14-3-3i A. thaliana, являющаяся аналогом соответствующего белка человека (14-3-3e), который является ингибитором AIF и играет ключевую роль на начальном этапе PCD у животных [95]. В условиях in vivo и in vitro показано, что цитохром с способен блокировать 14-3-3i и, таким образом, регулировать H2O2-индуцируемый процесс РСD в протопластах A. thaliana [95].
Следует также отметить, что появление цитохрома с в цитозоле клеток растений (и участие в процессе PCD), по-видимому, возможно без открытия p-mPTP и набухания митохондрий. В литературе есть данные, указывающие на возможность индукции поры во внешней мембране митохондрий растений с участием порообразующих белков типа BAX [77]. В частности, показано, что гетерологическая экспрессия животного белка BAX индуцировала PCD в растениях A. thaliana, которая подавлялась суперэкспрессией его ингибитора − BI-1 (BAX Inhibitor 1) [96]. Постулируется, что BI-1 является эволюционно консервативным белком и участвует в регуляции процесса PCD у животных и растений [77, 97]. С учетом вышеприведенных данных о множественности мишеней цитохрома с, эти результаты, на наш взгляд, подтверждают возможность существования у растений механизма PCD, являющегося близким аналогом классического апоптоза. Этот механизм включает образование поры во внешней мембране органелл с участием Bcl-2-подобных белков, а также формирование апоптосомо-подобного комплекса после выхода цитохрома с и других проапоптозных белков из митохондрий в цитозоль [75, 77, 98].
Как уже отмечалось выше, индукция mРТР в состоянии низкой проводимости в митохондриях животных может использоваться в качестве механизма, обеспечивающего быстрый выход из матрикса ионов Са2+, благодаря чему они способны играть ключевую роль в гомеостатировании уровня этого катиона в цитозоле клеток, принимать активное участие в Са2+-сигналинге, а также защищать органеллы от критического повышения уровня Са2+ и АФК в матриксе [22, 30, 99]. К сожалению, для митохондрий растений этот вопрос остается малоизученным, хотя нами было показано, что пермеабилизация внутренней мембраны митохондрий семядолей люпина, вследствие открытия t-mРТР, сопровождалась довольно быстрым выходом из матрикса накопленного Ca2+ [54]. Тем не менее, эта скорость была существенно ниже, чем в присутствии Са2+-ионофора (А23), а, кроме того, выход Са2+ мог быть связан с активацией или демаскированием (в результате ингибирования энергозависимого импорта Cа2+) других транспортных механизмов, например, Ca2+/Na+- или Са2+/Н+-антипортеров, катализирующих экспорт этого катиона из митохондрий животных и растений [96, 100]. Поэтому, для выяснения возможной функции t-mРТР в митохондриях растений в качестве Cа2+-канала, или разобщающего канала, в случае транспорта небольших катионов, необходимы дополнительные исследования.
Суммируя изложенные в обзоре материалы следует отметить, что, несмотря на существенный прогресс, достигнутый к настоящему времени в изучении mРТР в митохондриях животных, “белые пятна” все еще остаются, как в отношении ее структуры, так и механизмов регуляции активности. Однако гораздо более загадочными в настоящее время представляется регуляция функционирования и физиологическая роль mРТР в митохондриях растений, если априори признать ее строение тождественным с аналогом у животных [55]. Действительно, в литературе не стихают споры относительно существования AL-PCD у растений, даже с учетом его специфики, что создает проблему с определением функции mРТР. Как уже отмечалось, в последнее время в качестве основного пути PCD у растений рассматриваются и наиболее активно исследуются процессы аутофагии, а также ее селективной формы – митофагии [101, 102]. Однако следует отметить, что приведенные выше примеры не противоречат тому, что различные типы PCD, включая аутофагию, имеют место у растений в ходе их нормального развития и в стрессовых условиях. Значение аутофагии (митофагии) у растений, как и у животных или дрожжей заключается, либо в поддержании жизнедеятельности клетки путем удаления поврежденных молекул и митохондрий, путем заключения их в ограниченные двойной мембраной везикулы – аутофагосомы, препятствуя выходу из них белков инициирующих РСD, либо в индукции ее гибели, в том случае, если повреждение указанных клеточных структур достигло критического масштаба [102–104]. Другими словами, аутофагия может быть как ингибитором, так и активатором процесса PCD у растений. Более того, не исключено, что различные типы PCD протекают в клетках растений последовательно или параллельно и, например, митофагия может быть первичным ответом клетки на появление поврежденных митохондрий, характеризующихся повышенным образованием АФК и потерей m∆Ψ вследствие индукции mРТР [102, 105]. Показано, например, что при образовании перфораций в листьях Aponogeton madagascariensis в ходе PCD, идущего по пути мега-аутофагии, на раннем этапе в клетках были обнаружены многочисленные везикулы, содержащие агрегаты поврежденных, лишенных m∆Ψ митохондрий, которые мигрировали в вакуоли на позднем этапе PCD. При этом процесс перфорации листьев существенно замедлялся при обработке растений ЦсА [98, 106, 107].
ЗАКЛЮЧЕНИЕ
Таким образом, анализируя имеющиеся в литературе данные, можно заключить, что в ходе роста и развития растений в нормальных и стрессовых условиях митохондрии, будучи центром энергетического и пластического обмена, играют ключевую роль в процессах восприятия, интеграции и амплификации сигналов, поступающих в клетку различными путями и выступают в качестве основного регулятора процесса PCD независимо от его типа. На это указывает сходство многих регуляторов и сигналов, индуцирующих различные пути РCD: увеличение уровня АФК, АФА и концентрации ионов Ca2+ в цитозоле и матриксе митохондрий, деполяризация внутренней мембраны митохондрий, увеличение концентрации стрессовых фитогормонов и т.д. Об этом свидетельствует и неоднократно доказанная экспериментально консервативность первичных этапов индукции РСD в клетках животных и растений, включая диссипацию m∆Ψ, вследствие индукции mРТР, выход из органелл цитохрома с, а также обращение этого процесса под влиянием ЦсА. Было бы удивительно, если бы митохондрии, способные адекватно и системно реагировать на изменения метаболизма в ходе нормального развития растений, а также на действие различных неблагоприятных факторов среды, поддерживая жизнедеятельность клетки, были исключены из участия в программах, направленных на упорядоченный разбор клеточных структур в ходе ее естественной или стресс-индуцируемой гибели. Конечно, для выяснения конкретных механизмов, обеспечивающих взаимосвязь между нарушением функционирования митохондрий под влиянием внешних и внутренних факторов, и индукцией разных типов PCD у растений необходимы дополнительные исследования.
Работа выполнена при финансовой поддержке Министерства образования и науки Российской Федерации в рамках государственного задания (номер темы 121040800153-1 “Механизмы адаптации растений к факторам аридизации глобального климата и антропогенному загрязнению окружающей среды”).
Авторы заявляют об отсутствии конфликта интересов. Настоящая работа не содержит каких-либо исследований с участием людей и животных к качестве объектов исследования.
Список литературы
Ott M., Gogvadze V., Orrenius S., Zhivotovsky B. Mitochondria, oxidative stress and cell death // Apoptosis. 2007. V. 12. P. 913. https://doi.org/10.1007/s10495-007-0756-2
Giorgi C., Marchi S., Pinton P. The machineries, regulation and cellular functions of mitochondrial calcium // Nat. Rev. Mol. Cell Biol. 2018. V. 19. P. 713. https://doi.org/10.1038/s41580-018-0052-8
Sies H., Jones D.P. Reactive oxygen species (ROS) as pleiotropic physiological signalling agents // Nat. Rev. Mol. Cell Biol. 2020. V. 21. P. 363. https://doi.org/10.1038/s41580-020-0230-3
Hansen K.G., Herrmann J.M. Transport of proteins into mitochondria // Protein J. 2019. V. 38. P. 330. https://doi.org/10.1007/s10930-019-09819-6
Giacomello M., Pyakurel A., Glytsou C., Scorrano L. The cell biology of mitochondrial membrane dynamics // Nat. Rev. Mol. Cell Biol. 2020. V. 21. P. 204. https://doi.org/10.1038/s41580-020-0210-7
Rosencrans W.M., Rajendran M., Bezrukov S.M., Rostovtseva T.K. VDAC regulation of mitochondrial calcium flux: from channel biophysics to disease // Cell Calcium. 2021. V. 94:102356. https://doi.org/10.1016/j.ceca.2021.102356
Hunter D.R., Haworth R.A. The Ca2+-induced membrane transition in mitochondria. I. The protective mechanisms // Arch. Biochem. Biophys. 1979. V. 195. P. 453. https://doi.org/10.1016/0003-9861(79)90371-0
Haworth R.A., Hunter D.R. The Ca2+-induced membrane transition in mitochondria. II. Nature of the Ca2+ trigger site // Arch. Biochem. Biophys. 1979. V. 195. P. 460. https://doi.org/10.1016/0003-9861(79)90372-2
Hunter D.R., Haworth R.A. The Ca2+-induced membrane transition in mitochondria. III. Transitional Ca2+ release // Arch. Biochem. Biophys. 1979. V. 195. P. 468. https://doi.org/10.1016/0003-9861(79)90373-4
Halestrap A.P. What is the mitochondrial permeability transition pore // J. Mol. Cell Cardiol. 2009. V. 46. P. 821. https://doi.org/10.1016/j.yjmcc.2009.02.021
Bernardi P., Krauskopf A., Basso E., Petronilli V., Blalchy-Dyson E., Di Lisa F., Forte M.A. The mitochondrial permeability transition from in vitro artifact to disease target // FEBS J. 2006. V. 273. P. 2077. https://doi.org/10.1111/j.1742-4658.2006.05213.x
Bernardi P., Rassola A., Forte M., Lippe G. The mitochondrial permeability transition pore: channel formation by F-ATP syntase, interaction in signal transduction, and role in pathophysiology // Physiol. Rev. 2015. V. 95. P. 1111. https://doi.org/10.1152/phyrev.00001.2015
Bernardi P., Carraro M., Lippe G. The mitochondrial permeability transition: resent progress and open questions // FEBS J. 2022. V. 289. P. 7051. https://doi.org/10.1111/febs.162554
Giorgio V., Guo L., Bassot C., Petronilli V., Bernardi P. Calcium and regulation of the mitochondrial permeability transition // Cell Calcium. 2018. V. 70. P. 56. https://doi.org/10.1016/j.ceca.2017.05.004
Baines C.P. Loss of the cyclophilin D reveals a critical role for mitochondrial permeability transition in cell death // Nature. 2005. V. 434. P. 658. https://doi.org/10.1038/nature03434
Kroemer G., Galluzzi L., Brenner C. Mitochondrial membrane permeabilization in cell death // Physiol. Rev. 2007. V. 87. P. 99. https://doi.org/10.1152/physrev.00013.2006
Tajeddine N. How do reactive oxygen species and calcium trigger mitochondrial membrane permeabilization? // Biochim. Biophys. Acta. 2016. V. 1860 P. 1079. https://doi.org/10.1016/j.bbagen.2016.02.013
Bonora M., Giorgi C., Pinton P. Molecular mechanisms and consequences of mitochondrial permeability transition // Nat. Rev. Mol. Cell Biol. 2022. V. 23. P. 266. https://doi.org/10.1038/s41580-021-00433-y
Kanno T., Sato E.E., Muranaka S., Fujita H., Fujiwara T., Utsumi T., Inoue M., Utsumi K. Oxidative stress underlies the mechanism for Ca2+-induced permeability transition of mitochondria // Free Radic. Res. 2004. V. 38. P. 27. https://doi.org/10.1080/10715760310001626266
Martinez-Reyes I., Cuezva J.M. The H+-ATP synthase: a gate to ROS-mediated cell death ore survival // Biochim. Biophys. Acta. 2004. V. 1837. P. 1099. https://doi.org/10.1016/j.bbabio.2014.03.10
Bernardi P. The mitochondrial permeability transition pore: a mystery solved? // Front. Physiol. 2013. V. 4. P. 95. https://doi.org/10.3389/fphys.2013.00095
Ichas F., Mazat J.P. From calcium signaling to cell death: two conformations for the mitochondrial permeability transition pore. Switching from low– to high–conductance state // Biochim. Biophys. Acta. 1998. V. 1366 P. 33. https://doi.org/10.1016/S0005-2728(98)00119-4
Petronilli V., Miotto G., Canton M., Brini M., Colonna R., Bernardi P., Di Lisa F. Transient and long-lasting openings of the mitochondrial permeability transition pore can be monitored directly in intact cells by changes in mitochondrial calcein fluorescence // Biophys. J. 1999. V. 76. P. 725. https://doi.org/10.1016/S0006-3495(99)77239-5
Newmeyer D.D., Ferguson-Miller S. Mitochondria: releasing power for life and unleashing the machineries of death // Cell. 2003. V. 112 P. 481. https://doi.org/10.1016/S0092-8674(03)00116-8
Vianello A., Casolo V., Petrussa E., Peresson C., Patui S., Bertolini A., Passamonti S., Braidot E., Zancani M. The mitochondrial permeability transition pore (PTP) — an example of multiple molecular exaptation? // Biochim. Biophys. Acta. 2012. V. 1817. P. 2072. https://doi.org/10.1016/j.bbabio.2012.06.620
Yoon Y., Lee H., Federico M., Sheu S.-S. Non-conventional mitochondrial permeability transition: its regulation by mitochondrial dynamics // Biochim. Biophys. Acta. 2023. V. 1864:148914. https://doi.org/10.1016/j.bbabio.2022.148914
Leung A.W.C., Halestrap A.P. Recent progress in elucidating the molecular mechanism of the mitochondrial permeability transition pore // Biochim. Biophys. Acta Bioenerg. 2008. V. 1777. P. 946. https://doi.org/10.1016/j.bbabio.2008.03.009
Boyman L., Coleman A.K., Zhao G., Wescott A.P., Joca H.C., Greiser B.M., Karbowski M., Ward C.W., Lederer W.G. Dynamics of the mitochondrial permeability transition pore: transient and permanent opening events // Arch. Biochem. Biophys. 2019. V. 666. P. 31. https://doi.org/10.1016/j.abb.2019.03.016
Hüser J., Blatter L.A. Fluctuations in mitochondrial membrane potential caused by repetitive gating of the permeability transition pore // Biochem J. 1999. V. 343. P. 311. https://doi.org/10.1042/bj3430311
Bernardi P., Von Stockum S. The permeability transition pore as a Ca2+-release channel: new answers to an old question // Cell Calcium. 2012. V. 52. P. 22. https://doi.org/10.1016/j.ceca.2012.03.004
Crompton M. The mitochondrial permeability transition pore and its role in cell death // J. Biochem. 1999. V. 341. P. 233. https://doi.org/10.1042/bj3410233
Woodfield K., Rück A., Brdiczka D., Halestrap A.P. Direct demonstration of a specific interaction between cyclophiline-D and the adenine nucleotide translocase confirms their role in the mitochondrial permeability transition // Biochem. J. 1998. V. 336. P. 287. https://doi.org/10.1042/bj3360287
Kokoszka J.E. The ADP/ATP translocator is not essential for the mitochondrial permeability transition pore // Nature. 2004. V. 427. P. 461. https://doi.org/10.1038/nature02229
Gutiérrez-Aguilar M. Genetic manipulation of the cardiac mitochondrial phosphate carier does not affect permeability transition // J. Mol. Cell Cardiol. 2014. V. 72. P. 316. https://doi.org/10.1016/j.yjmcc.2014.04.008
Giorgio V., Von Stockum S., Antoniel M., Fabbro A., Fogolari F., Forte M., Glick G.D, Petronilli V., Zoratti M., Szabó I., Lippe G., Bernardi P. Dimers of mitochondrial ATP synthase form the permeability transition pore // Proc. Natl. Acad. Sci. USA. 2013. V. 110. P. 5887. https://doi.org/10.1073/pnas.1217823110
Alavian K.N., Beutner G., Lazrove E., Sacchetti S., Park H.A., Licznerski P., Li H., Nabili P., Hockensmith K., Graham M., Potter G.A. Jr., Jonas E.A. An uncoupling channel with the c–subunit ring of the F1F0–ATP synthase is the mitochondrial permeability transition pore // Proc. Natl. Acad. Sci. USA. 2014. V. 111 P. 10580. https://doi.org/10.1073/pnas.1401591111
Bonora M., Morganti C., Morciano G., Pedriali G., Lebiedzinska-Arciszewska M., Aquila G., Giorgi C., Rizzo P., Campo G., Ferrari R., Kroemer G., Wieckowski M.R., Galluzzi L., Pinton P. Mitochondrial permeability transition involves dissociation of F1FO ATP synthase dimers and C-ring conformation // EMBO Rep. 2017. V. 18. P. 1077. https://doi.org/10.15252/embr.201643602
Galber C., Minervini G., Cannino G., Boldrin F., Petronilli V., Tosatto S., Lippe G., Giorgio V. The f subunit of human ATP synthase is essential for normal mitochondrial morphology and permeability transition // Cell Rep. 2021. V. 35. P. 109. https://doi.org/10.1016/j.celrep.2021.109111
Giorgio V., Burchel V., Schiavone M., Bassot C., Minervini G., Petronilli V., Argenton F., Forte M., Tosatto S., Lippe G., Bernardi P. Ca2+ binding to F- ATP synthase beta subunit triggers the mitochondrial permeability transition // EMBO Rep. 2017. V. 18. P. 1065. https://doi.org/10.15252/embr.201643354
Karch J., Bround M.J., Khalil H., Sargent M.A., Latchman N., Terada N., Peixoto P.M., Molkentin J.D. Inhibition of mitochondrial permeability transition by deletion of the ANT family and CypD // Sci. Adv. 2019. V. 5. eaaw4597. https://doi.org/10.1126/sciadv.aaw4597
Neginskaya M.A., Solesio M.E., Berezhnaya E.V., Amodeo G.F., Mnatsakanyan N., Jonas E.A., Pavlov E.V. ATP synthase c-subunit-deficient mitochondria have a small cyclosporine a-sensitive channel, but lack the permeability transition pore // Cell Rep. 2019. V. 26. P. 11. https://doi.org/10.1016/j.celrep.2018.12.033
Carrer A., Tommasin L., Šileikytė J., Ciscato F., Filadi R., Urbani A., Forte M., Rasola A., Szabò I., Carraro M., Bernardi P. Defining the molecular mechanisms of the mitochondrial permeability transition through genetic manipulation of F-ATP synthase // Nat. Commun. 2019. V. 12:4835. https://doi.org/10.1038/s41467-021-25161-x
Vianello A., Macri F., Braidot E., Mokhova E.N. Effect of cyclosporin A on energy coupling in pea stem mitochondria // FEBS Lett. 1995. V. 371. P. 258. https://doi.org/10.1016/0014-5793(95)00897-i
Curtis M.J., Wolpert T.J. The victorine induced mitochondrial permeability transition precedes cell shrinkage and biochemical markers of cell death, and shrinkage occurs witgout of membrane integrity // Plant J. 2004. V. 38. P. 244. https://doi.org/10.1111/j.1365-313X.2004.02040.x
Fortes F., Castilho R.F., Catisti R., Carnieri E.G.S., Vercesi A.E. Ca2+ -induces a cyclosporin A-insensitive permeability transition pore in isolated potato tuber mitochondria mediated by reactive oxygen species // J. Bioenerg. Biomembr. 2001. V. 33. P. 43. https://doi.org/10.1023/a:1005672623709
Arpagaus S., Rawyler A., Braendle R. Occurrence and characteristics of the mitochondrial permeability transition in plants // J. Biol. Chem. 2002. V. 277. P. 1780. https://doi.org/10.1074/jbc.M109416200
Virolainen E., Blokhina O., Fagerstedt K. Ca2+-induced high amplitude swelling and cytochrome c release from wheat (Triticum aestivum L.) mitochondria under anoxic stress // Ann. Bot. 2002. V. 90. P. 509. https://doi.org/:10.1093/aob/mcf221
Tiwari B.S., Belenghi B., Levine A. Oxidative stress increased respiration and generation of reactive oxygen species, resulting in ATP depletion, opening of mitochondrial permeability transition, and programmed cell death // Plant Physiol. 2002. V. 128. P. 1271. https://doi.org//10.1104/pp.010999
Morimoto S., Tanaka Y., Sasaki K., Tanaka H., Fukamizu T., Shoyama Y., Shoyama Sh., Taura F. Identification and characterization of cannabinoids that induce cell death through mitochondrial permeability transition in Cannabis leaf cells // J. Biol. Chem. 2007. V. 282. P. 20739. https://doi.org/10.1074/jbc.M700133200
Shugaev A.G., Butsanets P.A., Shugaeva N.A. Salicylic acid induces the proton conductance in the inner mitochondrial membrane of lupine cotyledons // Russ. J. Plant Physiol. 2016. V. 63. P. 727. https://doi.org/10.1134/S1021443716060091
Romano P.G.N., Horton P., Gray J. The Arabidopsis cyclophilin gene family // Plant Physiol. 2004. V. 134. P. 1268. https://doi.org/10.1104/pp.103022160
Mainali H.R., Chapman P., Dhaubhadel S. Genome-wide analysis of cyclophilin gen family in soybean (Glycine max) // BMC Plant Biol. 2014. V. 14. P. 282. https://doi.org/10.1186/s12870-014-0282-7
De Coll V., Petrussa E., Casolo U., Braidot E., Filippi A., Peresson C., Patui S., Bertolini A., Giorgio V., Checchetto V., Vianello A., Bernardi P., Zancani M. Properties of the permeability transition of pea stem mitochondria // Front. Physiol. 2018. V. 9:1626. .https://doi.org/10.3389/fphys.2018.01626
Shugaev A.G., Butsanets P.A., Shugaeva N.A. Ca2+-dependent regulation of proton permeability of the inner membrane in the mitochondria of lupine cotyledons // Russ. J. Plant Physiol. 2023. V. 70: 73. https://doi.org/10.1134/S1021443723700127
Zancani M., Casolo V., Petrussa E.,Peresson C., Patui S., Bertolini A., De Coll V., Draidot E., Boscutti., Vianello A. The permeability transition in plant mitochondria: the missing link // Front. Plant. Sci. 2015. V. 6. P. 1120. https://doi.org/10.3389/fpls.2015.01120
Vlot C.A., Depsey D.A., Klessig D.F. Salicylic acid a multifaceted hormone to combat disease // Annu. Rev. Phytopathol. 2009. V. 47. P. 177. https://doi.org/10.1146/annualrev.phyto.050908.135202
Nie S., Yue H., Zhou J., Xing D. Mitochondrial-derived reactive oxygen species play a pivotal role in the salicylic acid signaling in Arabidopsis thaliana // Plos One. 2015. V. 10. e0119853. https://doi.org/10.1371/journal.pone.0119853
Belt K., Huang S., Thatcher L.F., Casarotto H., Singh K.B., Van Aken O., Millar A.H. Salicylic acid-dependent plant stress signaling via mitochondrial succinate dehydrogenase // Plant Physiol. 2017. V. 173. P. 2029. https://doi.org/10.1104/pp.1600060
Butsanets P.A., Shugaeva N.A., Shugaev A.G. Effect of melatonin and salicylic acid on ROS generation by mitochondria of lupine seedlings // Russ. J. Plant Physiol. 2021. V. 68. P. 745. https://doi.org/10.1134/S1021443721040038
Alvares M.E. Salicylic acid in the machinery of hypersensitive cell death and disease resistance // Mol. Cell Biol. 2000. V. 44. P. 499. https://doi.org/10.1023/a:1026561029533
Poore P. Effects of salicylic acid on the metabolism of mitochondrial reactive oxygen species in plants // Biomolecules. 2020. V. 10. P. 341. https://doi.org/10.3390/biom10020341
Battaglia V., Salvi M., Toninello A. Oxidative stress is resposible for mitochondrail permeability tsansition induction by salicylate in liver mitochondria // J. Biol. Chem. 2005. V. 280. P. 33864. https://doi.org/10.1074/jbc.M502391200
Arnao M.B., Hernandes-Ruis J. Functions of melatonin in plants: a review //J. Pineal. Res. 2015. V. 59. P. 133. https://doi.org/10.1111/jpi.12253
Khanna K., Bhardwaj R., Ahmad P., Reiter R. Phytomelatonin: a master regulator for plant oxidative stress management // Plant Physiol. Biochem. 2023. V. 196. P. 260. https://doi.org/10.1016/j.plaphy.2023.01.035
Hu C.-H., Zheng Y., Tong C.-L., Zhang D.-J. Effects of exogenous melatonin on plant growth, root hormones and photosynthetic characteristics of trifoliate orange subjected to salt stress // Plant Growth Regul. 2022. V. 97. P. 551. https://doi.org/10.1007/s10725-022-00814-z
Kobylinska A., Reiter R., Postmyk M. Melatonin protects cultured tobacco cells against lead-induced cell death via inhibition of cytochrome c translocation // Front. Plant Sci. 2017. V. 14: 1560. https://doi.org/10.3389/fpls.2017.01560
Wang P., Sun X., Wang N., Tan D.-X., Ma F. Melatonin enhances the occurrence of autophagy induced by oxidative stress in Arabidopsis seedlings // J. Pineal. Res. 2015. V. 58. P. 479. https://doi.org/10.1111/jpi.12233
Hibaoui Y., Roulet E., Ruegg U.T. Melatonin prevents oxidative stress-mediated mitochondrial permeability transition and death in skeletal muscle cells // J. Pineal Res. 2009. V. 47. P. 238. https://doi.org/j.1600-079X.2009.00707
Jou M.-J., Peng T.-I., Reiter R.J. Protective stabilization of mitochondrial permeability transition and mitochondrial oxidation during mitochondrial Ca2+ stress by melatonin’s cascade metabolites C3-OHM and AFMK in RBA1 astrocytes // J. Pineal Res. 2018. V. 66. e12538. https://doi.org/10.1111/jpi.12538
Van Doom W.G., Woltering E. Many ways to exit? Cell death categories in plants // Trends Plant Sci. 2005. V. 10. P. 117. https://doi.org/10.1016/j.tplants.2005.01.006
Wang P., Mugume Y., Bassman D.C. New advaces in autophagy in plants: regulation, selectivity and function // Sem. Cell Develop. Biol. 2018. V. 80. P. 133. https://doi.org/10.1016/j.semcdb.2017.07.018
Bock F.J., Tait S.W. Mitochondria as multifaceted regulator of cell death // Nat. Rev. Mol. Cell Biol. 2020. V. 21. P. 85. https://doi.org/10.1038/s41580-019-0173-8
Chu Q., Gu X., Zheng Q., Wang J., Zhu H. Mitochondrial mechanisms of apoptosis and necrosis in liver diseases // Anal. Cell. Pathol. 2021. V. 2021: 8900122. https://doi.org/10.1155/2021/8900122
Minina E.A., Dauphinee A.N., Ballhaus F., Gogvadze V., Stemenko A.P., Bozhkov P.V. Apoptosis is not conserved in plants as revealed by critical examination of model for plant apoptosis-like cell death // BMC Biology. 2021. V. 19: 100. https://doi.org/10.1185/s12915-021-01018-z
Dickman M., Williams B., Li Y., Figuieiredo P., Wolpert T. Reassessing apoptosis in plant // Nat. Plants. 2017. V. 3. P. 773. https://doi.org/10/1038/s4147-017-0020-x
Jones A. Does the plant mitochondrion integrate cellular stress and regulate programmed cell death? // Trends Plant Sci. 2000. V. 5. P. 225. https://doi.org/10.1016/s1360-1380(00)01605-8
Vianello A., Zancani M., Peresson C., Petrussa E., Casolo V., Krajnakova J., Patui S., Braidot E., Macri F. Plant mitochondrial pathway leading to programmed cell death // Physiol. Plant. 2007. V. 129. P. 242. https://doi.org/10.1111/j.1399-3054.2006.00767.x
Reape T.J., McCabe P.F. Apoptotic-like regulation of programmed cell death in plants // Apoptosis. 2010. V. 15. P. 249. https://doi.org/10.1007/s10495-009-0447-2
Van Aken O., Van Breusegem F. Licensed to kill: mitochondria, chloroplasts, and cell death // Trends Plant Sci. 2015. V. 20. P. 754. https://doi.org/10.1016/j.tplants.2015.08.002
Gutierrez-Aguilar M. Mitochondrial calcium transport and permeability transition as rational targets for plant protection // Biochim. Biophys. Acta. 2020. V. 1861. P. 148. https://doi.org/10.1016/j.bbabio.2020.148288
Yu X.H., Perdue T.D., Heimer Y.M., Jones A.M. Mitochondrial involvement in tracheary element programmed cell death // Cell Death Differ. 2002. V. 9. P. 189. https://doi.org/10.1038/sj.cdd.4400940
Vacca R.A., Valenti D., Bobba A., Merafina R.S., Passarella S., Marra E. Cytochrome c is released in a reactive oxygen species-dependent manner and is degraded via caspase-like proteases in tobacco Brigth-Yellow 2 cells en route to heat shock-induced cell death // Plant Physiol. 2006. V. 141. P. 208. https://doi.org/10.1104/pp.106.078683
Lin J., Wang Y., Wang G. Salt stress-induced programmed cell death in tobacco protoplasts is mediated by reactive by reactive oxygen species and mitochondrial permeability transition pore status // J. Plant Physiol. 2006. V. 163. P. 731. https://doi.org/10.1016/j.jplph.2005.06.016
Gao C., Xing D., Li L., Zang L. Implication of reactive oxygen species and mitochondrial disfunction in the early stages of programmed cell death induced by ultraviolet-C overexposure // Planta. 2008. V. 227. P. 755. https://doi.org/10.1007/s00425-007-0654-4
Stein J.C., Hansen G.M. Mannose induces an endonuclease responsible for DNA laddering in plant cells // Plant Physiol. 1999. V. 121. P. 71. https://doi.org/1104/pp.121.1.71
Yao N., Eisfelder B.J., Marvin J., Greenberg J.T. The mitochondria: an organelle commonly involved in programmed cell death in Arabidopsis thaliana // Plant J. 2004. V. 40. P. 1803. https://doi.org/10.1111/j.1365-313X.2004.02239.x
Garcia-Heredia L.M., Hervas M., De la Rosa M.A., Navaro J.A. Acetylsalicylic acid induced programmed death in Arabidopsis cell cultures // Planta. 2008. V. 228. P. 89. https://doi.org/10.1007/s00425-008-0721-5
Krause M., Durner J. Harpin inactivates mitochondria in Arabidopsis suspension cells // Mol. Plant Microbe Interact. 2004. V. 17. P. 131. https://doi.org/10.1094/MPMI.2004.17.2.131
Amirsadeghi S., Robson C., Vanlerberghe G.C. The role of the mitochondria in plant responses to biotic stress // Physiol. Plant. 2007. V. 129. P. 253. https://doi.org/10.1111/j.1399-3054.2006.00775.x
Mur L.A., Kenton P., Lloyd A.J., Ougham H., Prats E. The hypersensitive response: the centenary is upon us but much do me know? // J. Exp. Bot. 2008. V. 59. P. 501. https://doi.org/10.1093/jxb/erm239
Colombatti F., Gonzales D.H., Welchen E. Plant mitochondria under pathogen attack: a sigh of relief or a last breath? // Mitochondrion. 2014. V. 19. P. 238. https://doi.org/10/1016/j.mito.2014.03.006
Salvesen G.S., Hempel A., Coll N.S. Protease signaling in animal and plant-regulated cell death // FEBS J. 2016. V. 238. P. 2577. https://doi.org/10.1111/febs.13616
Buono A.R., Hudecek R., Nowack M.K. The roles of proteases during developmental programmed cell death in plant // J. Exp. Bot. 2019. V. 70. P. 2097. https://doi.org/10.1093/jxb/erz072
Martinez-Fabregas J., Diaz-Moreno I., Gonzales-Arzola K., Janocha S., Navarro J.A., Hervás M., Bernhardt R., Díaz-Quintana A., De la Rosa M.Á. New Arabidopsis thaliana cytochrome c partners: a look into the elusive role of cytochrome c in Programmed Cell Death in plants // Mol. Cell Proteomics. 2013. V. 12. P. 3666. https://doi.org/10.1074/mcp.M113.030692
Elena-Real C.A., Gonzalez-Arzola K., Peres-Mejias G., Diaz-Quintana A., Velazquez-Campoy A., Desvoyes B., Gutierez C., De la Rosa M.A., Diaz-Moreno I. Proposed mechanism for regulation of H2O2-induced programmed cell death in plants by binding cytochrome c to 14-3-3 proteins // Plant J. 2021. V. 106. P. 74. https://doi.org/10.1111/tpj.15146
Kawai-Yamada M., Ohori Y., Uchimiya H. Dissection of Arabidopsis Bax inhibitor -1 suppressing Bax-, hydrogen peroxide-, and salicylic acid-induced cell death // Plant Cell. 2004. V. 16. P. 21. https://doi.org/10.1105/tpc.014613
Watanabe N., Lam E. Recent advance in the study of caspase- ike proteases and Bax inhibitor 1 in plants: their possible role as regulator of programmed cell death // Mol. Plant Patol. 2004. V. 5. P. 65. https://doi.org/10.1111/1364-3703-2004-00206
Lord C.E.N., Gunawardena A.H.L.A.N. Programmed cell death in C. elegans, mammals and plants // Eur. J. Cell Biol. 2012. V. 91. P. 603. https://doi.org/10.1016/j.ejcb.2012.02.002
De Marchi E., Bonora M., Giorgi C., Pinton P. The mitochondrial permeability transition pore is a dispensable element for mitochondrial calcium efflux // Cell Calcium. 2014. V. 56. P. 1. https://doi.org/10.1016/j.ceca.2014.03.004
Carraretto L., Checchetto V., De Bortoli S., Formentin E., Costa A., Szabo I., Teardo E. Calcium flux across plant mitochondrial membranes: possible molecular players // Front. Plant Sci. 2016. V. 7: 354. https://doi.org/10.3389/fpls.2016.00354
Bozhkov P.V. Plant autophagy mechanisms and function // J. Exp. Bot. 2018. V. 69. P. 1281. https://doi.org/10.1093/jxb/ery070
Broda M., Millar A. H., Van Aken O. Mitophagy: a mechanism for plant growth and survival // Trend Plant Sci. 2018. V. 23. P. 434. https://doi.org/10.1016/j.plants.2018.02.010
Ren K., Feng L., Sun S., Zhuang X. Plant mitophagy in comparison to mammals: what is still missing? // Int. J. Mol. Sci. 2021. V. 22. P. 1236. https://doi.org/10.3390/ijms22031236
Kumar R., Reichert A.S. Common principles and specific mechanisms of mitophagy from yeast to humans // Int. J. Mol. Sci. 2021. V. 22. P. 4363. https://doi.org/10.3390/ijms22094363
Ye C., Zheng S., Jiang D., Lu J., Huang Z., Liu Z., Zhou H., Zhuang H., Li J. Initiation and execution of programmed cell death and regulation of reactive oxygen species in plants // Int. J. Mol. Sci. 2021. V. 22. P. 12942. https://doi.org/10.3390/ijms.222312942
Lord C.E.N., Weitman J.N., Lane S., Gunawardena A.H. Do mitochondria play a role in remodeling lace plant leaves during programmed cell death? // BMC Plant Biol. 2011. V. 11. P. 102. https://doi.org/10.1186/1471-2229-11-102
Weitman J., Lord E.N., Dauphinee, Gunawardena A.H. The pathway of cell dismantling during programmed cell death in lace plant (Aponogeton madagascariensis) leaves // BMC Plant Biol. 2012. V. 12. P. 115. https://doi.org/10.1186/1471-2229-12-115
Дополнительные материалы отсутствуют.
Инструменты
Физиология растений