Геоморфология и палеогеография, 2022, T. 53, № 5, стр. 162-172
СТРОЕНИЕ И ИСТОРИЯ ФОРМИРОВАНИЯ ДОЛИН ПРОРЫВА ВЕРХНЕЙ ВОЛГИ
А. О. Уткина 1, *, А. В. Панин 1
1 Институт географии РАН
Москва, Россия
* E-mail: utkina@igras.ru
Поступила в редакцию 14.06.2022
После доработки 29.06.2022
Принята к публикации 15.07.2022
- EDN: FXDLFX
- DOI: 10.31857/S0435428122050182
Аннотация
Несмотря на длительную историю изучения, история формирования долины верхней Волги представляет предмет дискуссий. Согласно наиболее распространенной в литературе модели, в максимум последнего оледенения в бассейне верхней Волги возникла система приледниковых озер, и долина Волги образовалась лишь 14–15 тыс. л. н. после спуска этих озер в результате образования участков прорыва у нынешних городов Тутаева и Плеса. Чтобы проверить эту гипотезу, мы попытались определить механизм формирования и возраст долин прорыва, используя метод люминесцентного датирования. Кроме того, для оценки одного из возможных механизмов образования приледниковых озер мы использовали модель гляциоизостатических деформаций. В долине прорыва у г. Плёс нами была обнаружена речная терраса, датированная позднемосковско-микулинским временем, что доказывает, что долина намного старше предполагаемого валдайского возраста. В долине не было обнаружено никаких признаков озерных отложений, а моделирование не показало такого влияния гляциоизостатических деформаций на бассейн, которое могло бы привести к образованию приледниковых озер. В связи с этим можно предположить, что валдайских приледниковых озер в долине не существовало. По-видимому, предыдущими исследователями за озерные отложения принимались широко развитые на склонах и дне долины делювиальные суглинки и эоловые алевриты, которые, по данным нашего датирования, действительно относятся к эпохе последнего оледенения. По данным моделирования, в последнюю ледниковую эпоху на изучаемый участок приходится формирование компенсационного приледникового вала. Его высоты было недостаточно для подпруживания Волги, но уменьшение уклона долины должно было вызвать направленную аккумуляцию в реке, что подтверждается наличием низкой речной террасы соответствующего возраста. В позднеледниковье разрушение вала вызвало врезание реки.
1. INTRODUCTION
With a length of 3.530 km and the drainage basin area of 1.361 million km2 (Tockner et al., 2009) the Volga is the largest river in Europe. Today the river is almost entirely regulated as it flows through Central Russia and into the Caspian Sea. The Volga has been a focus of geographic, geomorphologic and paleogeographic research for several decades, and many attempts to reconstruct its history have been made (Sidorchuk et al., 2009). Nevertheless, there is still much to uncover about how and when it was formed. Particularly this concerns the upstream part of the basin, as its evolution, though much studied, remains uncertain. The Volga River is traditionally divided into three unequal parts based on the major differences in its geological and geomorphological structure (Obedientova, 1977), and its upstream part is referred to as the Upper Volga (fig. 1). When compared to other parts of the valley, the amount of conducted research on the Upper Volga is significantly lesser.
Fig. 1.
Elevation map of the research area: digital elevation model (DEM) SRTM. Ice sheet extents are shown as blue dashed lines (vd – MIS 2 ice sheet extent (Astakhov et al., 2016), ms – MIS 6 ice sheet extent (Moskvitin, 1967)); red dots mark study sites (1 – Otmishchevo site, 2 – Pogorelka site, 3 – Plyos site).
Рис. 1. Схема района исследования. Основа – цифровая модель рельефа (ЦМР) SRTM. Границы оледенений показаны синими пунктирными линиями (vd – валдайское оледенение (MIS 2) (Astakhov et al., 2016), ms – московское (MIS 6) (Москвитин, 1967)); красными точками отмечены ключевые участки (1 – Отмищево, 2 – Погорелка, 3 – Плес).
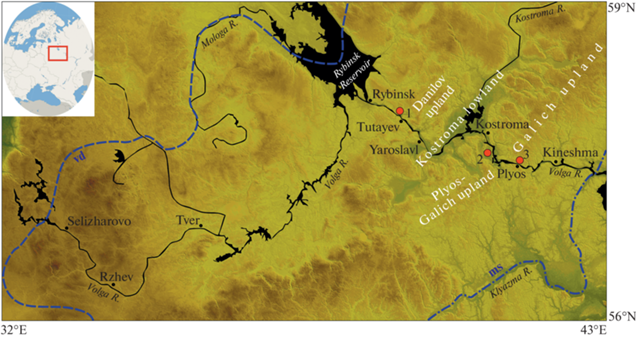
One of the key issues of the paleogeography of the Upper Volga is the formation of incision valleys since this process is associated with the river network restructuring. These incision valleys are narrow sections of the Upper Volga valley characterised by a deeply cut channel, high banks, and the absence of river terraces. Their formation is assumed to involve a rapidly occurred cutting through the local watershed. Since such valley sections usually indicate that the valley itself is relatively young, the researchers (Schukina, 1933; Mirchink, 1935; Kvasov, 1979) conclude that it joined the rest of the river system only recently, supposedly, in late Pleistocene.
Over the years, it had been established that the formation of the Upper Volga River must had been influenced by Pleistocene glaciations of the Northern Russian Plain. It is widely known that they played a major role in shaping the modern geomorphologic structure of the region (Kalm, 2012). Most researchers argue that the formation of the Upper Volga River occurred at the last stages of the Last Glaciation (called Valdai glaciation on the Russian plain) (Schukina, 1933; Kvasov, 1979). Since its basin was largely affected by the last glaciation event, the Upper Volga supposedly could have been one of the regions where proglacial lakes emerged during the Late Valdai (Bolshakova, 1963; Schik, Pisareva, 1998; Astakhov et al., 2016). This concept was first introduced by Kvasov (1979). According to his model, a major part of Upper Volga territory was covered by a large lake system that formed during the last glacial maximum (LGM, approx. 21 ka). The river itself was to appear only after this lake system had stopped existing. Presumably, it happened due to formation of an incision valley near the town of Plyos (fig. 1) about 14.5 ka (Kvasov, 1979). Kvasov does not go into detail on Tutaev incision valley’s mechanism of formation but concludes that it was formed when the waters of the proglacial lake cut through the Danilov upland around 15 ka.
This concept of the Upper Volga River’s emergence, through widely accepted, has not been thoroughly checked and lacks proper stratigraphical evidence. There are no direct determinations of the age of these events, as well as of the time of river valley adjustment. Many questions concerning the paleogeography of ice-dammed lakes are far from being resolved. Areas of flooding, elevation of the lake levels in relation to the thresholds of runoff, location, and chronology of overflow events, restructuring of valley systems – these issues have still been addressed mainly on the morphological basis (Mangerud et al., 2004). Virtually no geologically confirmed facts have still been published, i.e., reliably proved occurrence of deposits of the corresponding genesis and their age.
There is also one important aspect of the history of Pleistocene periglacial areas that could not had been considered by Kvasov in late 1970s. The formation of proglacial lakes in the Upper Volga basin could have been heavily influenced by glacial isostatic adjustment (GIA). One of the most important effects of GIA impact on the landscape development was the formation of large lithosphere uplifts located in the periglacial zones called glacial forebulges. Previous study of large rivers valleys in Europe, as well as the relief of the bottom of the North Sea and the English Channel, carried out by E.N. Bylinsky (1990), made it possible to outline the location of Late Valdai glacial forebulge around the Scandinavian ice sheet. According to Bylinsky’s model (Bylinski, 1990), the glacial forebulge of the last glaciation crossed the Upper Volga basin in close vicinity of the Plyos incision valley. One of the possible mechanisms for the proglacial lakes’ formation could involve slight inclination of the basin towards the glacier. This way, the part of the basin located within the glacial forebulge would be hypsometrically higher than its source. Such an incline of the basin surface would allow the waters to be dammed from both north (by the glacier) and south (by the forebulge). It is possible to determine the reliability of this mechanism by employing the GIA model (Spada, Melini, 2019).
This paper aims to demonstrate the results of recent studies that could provide a geochronological insight into the history of the Upper Volga. Our studies are focused mainly on the incision valleys, as their emergence might be the key event in the Upper Volga valley’s history. The main goal of the study was to determine the age of the Plyos and Tutayev incision valleys and the mechanism of their formation. Also, since their emergence is possibly connected with the formation of the Upper Volga proglacial lakes, we used a GIA model (Spada, Melini, 2019) to assess one of the ways of their possible formation. As this study concerns questions of possible existence of proglacial lakes on the Northern Russian Plain, it can provide new knowledge about them. It is widely known that outbursts and overflows of the large proglacial lakes through watersheds exerted impact over geomorphological landscapes and caused the paleogeographic events of regional and possibly global rank (Svendsen et al., 2004).
2. MATERIALS AND METHODS
During fieldwork 9 excavations were made in the key areas (7 boreholes made by mechanical drilling, and 2 sections). Mechanical drilling was conducted using the Mount 80 drilling rig. Based on the sedimentological description we made assumptions of the possible genesis of the sediments.
We employed Optically Stimulated Luminescence (OSL) as the main dating method. It is widely used for dating glaciofluvial (Thrasher et al., 2009) and fluvial (Wallinga, 2002) sediments and has proven to be able to provide reliable results for low-gradient river systems in central Russia (Panin et al., 2017), and in particular in the Volga system (Kurbanov et al., 2020). All samples were collected in opaque plastic tubes secured with foil on both ends. To collect the samples during mechanical drilling a sampler designed specifically for this purpose was used. The potentially light-exposed ends of the sample were used for dose rate measurements. A total of 25 samples was collected. Preliminary sample preparation was performed in Moscow, MSU, under orange LED lights (Sohbati et al., 2017), following the procedure described by (Kurbanov et al., 2020) and (Murray et al., 2021). All measurements were performed at the Nordic Laboratory for Luminescence Dating at Risø, Denmark, using multi-grain aliquots. Samples were measured in a Risø TL/OSL reader, model TLDA20, equipped with a calibrated beta source (dose rate 0.057–0.220 Gy/s) (Hansen et al., 2015, 2018). For quartz, the single aliquot regenerative dose protocol was applied to 10 mm diameter multi-grain (180–250 mm) quartz aliquots to estimate the equivalent dose (Murray, Wintle, 2000, 2003), with blue (470 ± 30 nm) light stimulation, and 260°C preheating for 10 s. Multi-grain (180–250 mm) feldspar aliquots (2 mm diameter) were measured using a post IR-IR protocol, with a preheat temperature of 250°C for 1 min, and stimulation with IR (870 nm) for 100 s while the aliquot was held at 50°C (IR50), followed by a further 100 s with the sample held at 290°C (pIRIR290) (Thomsen et al., 2008; Buylaert et al., 2012). Large multi-grain aliquots were employed as it was important to identify well-bleached samples; the average dose is then the most appropriate dose estimate, and for a given number of measurements, this is most precisely measured using large aliquots.
The samples were analysed for natural radionuclide concentrations using high-resolution gamma spectrometry (Murray et al., 1987). These concentrations were converted into dose rates using conversion factors listed by (Olley et al., 1996); a cosmic ray contribution was calculated according to (Prescott, Hutton, 1994), assuming the modern burial depth has applied throughout the lifetime of the site. Laboratory saturated water contents were measured.
Dating sediments of glaciofluvial and fluvial origin is often associated with an incomplete bleaching of grains in the transport process (Alexanderson, Murray, 2012). Using both quartz and feldspar signals, we expected to investigate the degree of bleaching of the quartz by comparing quartz OSL ages with feldspar IR and pIRIR ages (Murray et al., 2012).
GIA modelling was also employed to investigate possible GIA influence on the Upper Volga during the Late Pleistocene. We used the open-source program SELEN (version 4.0) (Spada, Melini, 2019) that simulates the glacial isostatic adjustment (GIA) process in response to the melting of the Late Pleistocene ice sheets. SELEN solves the gravitationally and topographically self-consistent sea level equation for a spherically symmetric Earth with linear viscoelastic rheology, while considering the migration of the shorelines and the rotational feedback on sea level. SELEN thus allows to model GIA in any given part of the world and study a broad range of its geophysical effects. To simulate the GIA effect, SELEN uses data on the spatio-temporal distribution of glacier loads extracted from the ICE-6G model (Argus et al., 2014; Peltier et al., 2015), as well as information about modern topography based on ETOPO1. Following the user guide distributed along with the source code (Spada, Melini, 2019) we ran the program in its standard configuration. Using the resulting data on the reconstruction of topography we were able to study the changes in topography under the GIA influence for three time periods (21 ka (LGM), 17.5 ka, 15 ka). Kvasov (1979) chose these exact time stamps to reconstruct the Upper Volga proglacial lakes. Using the GIS software (ArcMap 10.5) DEMs of the “paleo-topography” for the mentioned time periods were created which allowed us to directly determine the changes in topography caused by the GIA effect. For a visual demonstration of these changes in the Upper Volga basin, river profiles were constructed for each of the time slices and for the modern river and then compared. More detailed explanation of our modelling strategy can be found in our recent paper (Utkina, 2020).
3. RESULTS
Both incision valleys are in the Upper Volga valley section extending from Rybinsk reservoir to Plyos (fig. 1). In the Rybinsk-Yaroslavl region the river flows in a narrow valley while crossing the Danilov upland. The terrain is relatively hilly alternating in places with flat areas, typically 150 to 200 m above sea level (a.s.l.). The valley width varies from 1.5 to 2 km, and the channel width from 700 to 900 m, with the narrowest part located near Tutayev (Tutayev incision valley). Downstream from Yaroslavl the valley expands up to 20 km while flowing through the Kostroma lowland, and then narrows again to cut through the Plyos-Galich upland, forming the Plyos incision valley. Here, about 150 km downstream from Tutayev, the valley is only 600–700 m wide, with 40–60 m high steep slopes (around 20°) lining the channel. According to previous studies (Schukina, 1933; Bolshakova, 1963; Obedientova, 1977; Kvasov, 1979), there are no alluvial sediments found in the incision valleys, however some were discovered a few kilometres upstream from them. In this study we aimed to check if this pre-established view on the Upper Volga valley structure is true or not.
The resulting OSL equivalent doses, dose rates and ages are shown in tabl. 1 together with feldspar to quartz ratios. The general luminescence characteristics for the samples from the Upper Volga are reported in our previous paper (Utkina et al., 2022).
Table 1.
Quartz and feldspar doses, resulting ages and feldspar/quartz age ratios Таблица 1. Результаты люминесцентного датирования: дозы, итоговые возраста и их соотношения по кварцу и полевому шпату
Sample code |
Depth. cm | Equivalent dose, Gy | Age, ka | Quartz dose rate (Gy/ka)a | IR50/Q | pIRIR290/Q | Bb | ||||
---|---|---|---|---|---|---|---|---|---|---|---|
Quartz OSL | IR50 | pIRIR290 | Quartz OSL | IR50 | pIRIR290 | ||||||
19542–1 | 225 | 35 ± 1 | 37 ± 1 | 50 ± 2 | 20 ± 1 | 14 ± 1 | 20 ± 1 | 1.76 ± 0.1 | 0.7 ± 0.1 | 1.0 ± 0.1 | 3 |
19542–2 | 275 | 35 ± 1 | 37 ± 1 | 51 ± 2 | 18 ± 1 | 14 ± 1 | 19 ± 1 | 1.94 ± 0.1 | 0.8 ± 0.1 | 1.0 ± 0.1 | 3 |
19543–1 | 295 | 50 ± 2 | 66 ± 4 | 117 ± 13 | 28 ± 2 | 26 ± 2 | 46 ± 6 | 1.78 ± 0.08 | 0.9 ± 0.1 | 1.64 ± 0.2 | 3 |
19543–2 | 405 | 116 ± 5 | 189 ± 23 | 259 ± 40 | 64 ± 5 | 73 ± 9 | 100 ± 16 | 1.81 ± 0.09 | 1.14 ± 0.3 | 1.57 ± 0.4 | |
19543–3 | 525 | >200 | 389 ± 9 | 783 ± 66 | >90 | (130)c | (270) | 2.18 ± 0.10 | |||
19543–4 | 670 | >200 | 576 ± 44 | 809 ± 77 | >160 | 280 ± 20 | 390 ± 40 | 1.29 ± 0.06 | |||
19543–5 | 750 | >200 | 508 ± 30 | 701 ± 59 | >170 | 260 ± 20 | 360 ± 40 | 1.15 ± 0.06 | |||
19544–1 | 85 | 1.1 ± 1 | 19 ± 1 | 65 ± 1 | 0.57 ± 0.1 | 8 ± 1 | 26 ± 2 | 1.73 ± 0.08 | 13 ± 2 | 43 ± 5.7 | |
19544–2 | 90 | 1.7 ± 1 | 20 ± 1 | 63 ± 1 | 0.97 ± 0.1 | 8 ± 1 | 26 ± 1 | 1.68 ± 0.08 | 8 ± 1 | 25 ± 3.4 | |
19544–3 | 180 | 21 ± 1 | 25 ± 1 | 57 ± 1 | 9.2 ± 0.5 | 8 ± 1 | 18 ± 1 | 2.33 ± 0.11 | 0.9 ± 0.1 | 2.0 ± 0.2 | |
19544–4 | 210 | 19 ± 1 | 24 ± 1 | 55 ± 1 | 9.8 ± 0.6 | 9 ± 1 | 20 ± 1 | 1.99 ± 0.10 | 0.9 ± 0.1 | 2.1 ± 0.2 | |
19535–1 | 150 | >200 | 463 ± 14 | 835 ± 158 | >260 | 300 ± 10 | 540 ± 110 | 0.78 ± 0.03 | |||
19535–2 | 250 | >200 | 522 ± 36 | 976 ± 128 | >280 | 350 ± 30 | 660 ± 90 | 0.71 ± 0.03 | |||
19535–3 | 350 | >200 | 444 ± 17 | 740 ± 35 | >230 | 270 ± 20 | 440 ± 30 | 0.89 ± 0.04 | |||
19535–4 | 395 | >200 | 464 ± 22 | 899 ± 186 | >240 | 290 ± 20 | 560 ± 120 | 0.83 ± 0.04 | |||
19534–3 | 690 | 18 ± 1 | 24 ± 1 | 35 ± 2 | 14 ± 1 | 10 ± 1 | 14 ± 1 | 1.69 ± 0.09 | 0.9 ± 0.1 | 1.3 ± 0.1 | 3 |
19534–4 | 150 | 36 ± 1 | 35 ± 1 | 54 ± 1 | 16 ± 1 | 10 ± 1 | 16 ± 1 | 2.55 ± 0.13 | 0.7 ± 0.1 | 1.2 ± 0.1 | 3 |
19534–5 | 380 | 36 ± 1 | 32 ± 1 | 50 ± 1 | 14 ± 1 | 11 ± 1 | 17 ± 1 | 2.20 ± 0.11 | 0.7 ± 0.1 | 1.0 ± 0.1 | 3 |
19534–6 | 450 | 33 ± 1 | 33 ± 1 | 53 ± 1 | 11 ± 1 | 11 ± 1 | 17 ± 1 | 2.33 ± 0.12 | 0.8 ± 0.1 | 1.2 ± 0.1 | 3 |
010–5 | 80 | 111 ± 4 | 154 ± 17 | 255 ± 20 | 119 ± 10 | 83 ± 10 | 136 ± 14 | 0.93 ± 0.04 | 0.69 ± 0.1 | 1.15 ± 0.1 | 3 |
010–6 | 130 | 116 ± 5 | 163 ± 21 | 238 ± 15 | 108 ± 8 | 81 ± 12 | 118 ± 10 | 1.07 ± 0.04 | 0.75 ± 0.1 | 1.09 ± 0.1 | 3 |
010–1 | 470 | 62 ± 7 | 207 ± 20 | 453 ± 57 | 59.5 ± 8 | 100 ± 11 | 230 ± 32 | 1.04 ± 0.05 | 1.71 ± 0.3 | 3.85 ± 0.67 | |
010–2 | 730 | 167 ± 23 | 265 ± 25 | 376 ± 29 | 153 ± 24 | 131 ± 14 | 186 ± 17 | 1.09 ± 0.04 | 0.85 ± 0.2 | 1.21 ± 0.2 | 3 |
010–3 | 870 | 126 ± 6 | 137 ± 16 | 285 ± 7 | 124 ± 9 | 70.5 ± 9 | 146 ± 7.7 | 1.01 ± 0.04 | 0.57 ± 0.1 | 1.17 ± 0.1 | 3 |
010–4 | 890 | 155 ± 5 | 244 ± 17 | 362 ± 26 | 120 ± 7 | 109 ± 9 | 162 ± 14 | 1.30 ± 0.05 | 0.92 ± 0.1 | 1.36 ± 0.1 | 3 |
3.1. Tutayev incision valley. To examine the valley structure close to Tutayev incision valley, we studied a site near Otmishchevo village (located 4 km upstream from Tutayev (fig. 1, site 1)), where previous researchers identified three Volga terraces (Arslanov et al., 1972). We drilled 5 boreholes and studied one section in the proposed terrace levels, comprising the resulting data into a profile (fig. 2). The first borehole (19541) is in a depression on a gently sloping surface, previously interpreted as the third Volga River terrace. Up to a depth of 4.5 m and below the core is comprised of reddish-brown loam with sporadic grus. From 3.5 m, it becomes brick-red with large fragments of ground metamorphic rocks. Above 2.2 m it is overlain by a medium silt loam. Overall sequence displays slope wash deposits, and no sediments of alluvial origin are found.
The second borehole was placed closer to the edge of the same surface (19542, fig. 2). At 3.0–4.5 m and below, there is a heavy brick-red loam with rocks (till). Below 2.5 m it is covered by fine sand with layers of 2–3 cm thick loam. Fine-grained sand with a thin horizontal layering follows from 1.9 m. The upper 0.6 m reveal a light red-brown silty loam. 2 samples were taken for OSL dating: at 2.0–2.5, and at 2.5–3.0 m. The sediments date back to Late Valdai (18–20 ka, MIS 2). The age was determined with high reliability since the quartz and feldspar ratios are 1.01–1.04 (pIRIR290/Q) and 0.7–0.8 (IR50/Q) (tabl. 1). The described sediment composition allows us to determine their slope genesis. Two additional boreholes were made here to trace the top of a till layer (19 545 and 19 546, fig. 2). The depth of the top changed from 3 to 7.7 m. The upper part of both revealed sequences is seemingly similar to the borehole 19542.
The next borehole (19 543) is located down the slope, near the edge of a lower terrace level (105 m a.s.l.). The sequence starts with a till layer at the bottom. The next 6.8 m are comprised of a loamy sequence, probably water lain, with several coarser layers (at 7, 4, and 3 m deep). Samples for OSL dating were taken from depths of 7.0–7.5 m (clayey sand with debris), 6.6–6.9 m (sandy loam), 5.0–5.5 m (silty loam). This type of sediment cannot be directly attributed to one distinct deposition process, but given the abundance of fine-grained material, it was probably deposited in relatively calm water conditions with coarser layers corresponding to periods of faster water flow. The upper part of the section (1 m) is comprised of fine, probably aeolian, sands. Based on the ages, this section has two distinct parts: the upper 4 m appears to be from the last glacial period, and below that the sediments are much older, around 200–300 ka.
Down the slope the first floodplain terrace of the Volga River was discovered. The terrace ledge is sharp and clear at the confluence of the Dubenka River with the Volga. The ledge is steep, the height is about 8 m (91 m a.s.l.) above the Volga summer water level. A terrace section (19 544) revealed reddish-brown layered sandy loam with fine-grained sand and sporadic pebbles continuing up to 1.8 m deep. Above 1.80 m, multiple layers (1 to 100 mm thick) of fine-grained sands alternating with sandy loam were observed. Below 1.5 m, the layers are thinner, 1–5 mm thick, with the increased number of loamy interlayers. Visible horizontal layering is characteristic for the entire sequence. Samples for OSL were collected at 0.75–0.80, 0.80–0.85, 1.80–1.85, 2.20–2.25 m. The resulting ages date back to the Holocene: the upper ones are around 1 ka, and the lower ones – around 10 ka. The quartz and feldspar ratios indicate the average reliability of the date (tabl. 1).
3.2. Plyos incision valley. Field studies in Plyos incision valley were conducted both inside the valley and 30 km upstream from it, where previous studies had described the terraces as particularly well defined (Bolshakova, 1963). The latter site (Pogorelka) is located ~100 km downstream from Tutayev (fig. 1, site 2). Bolshakova (1963) identified two terraces in this part of the Volga valley, with altitudes 110 m a.s.l. (a conjugate terrace of Volga and Kostroma Lake) and 100 m a.s.l. Our chosen site revealed what appeared to be 3 terraces at 90, 100 and 112 m a.s.l. We studied the sediment composition of the upper (oldest) terrace (19 535, 112 m a.s.l.) and the middle terrace (19 534, 100 m a.s.l.) (fig. 3). The oldest supposed terrace is composed of a 7.5 m-thick sandy and loamy sequence resting on loamy till layer with boulders. The sediment layer covering the till is made of fine loamy and silty layers, further up it is replaced by 1m of fine sand (sampled for OSL at 3.8 and 3.5 m deep) with visible horizontal layering. The next 2 m are represented by coarser sand with rare gravel and pebbles (sampled for OSL at 2.5 and 1.5 m). Finally, this layer is followed by 0.5 m of medium-grained sand and silt alternation and then by 0.5 m-thick sequence made up of loam. OSL dating revealed that these sediments are again much older than Late Valdai, around 400 ka and even up to 660 ka.
The second proposed terrace (19 534, fig. 3) revealed a loamy till layer at a depth of 8 m, and further up it is replaced by fine-grained clayey sand. At 7.5 m, there is a silty loamy layer, and at 5.5 m – a silty layer with a clear horizontal layering. In the upper 2.6 m, the core is represented by silt with a clayey interlayer at 2.2–2.6 m. OSL samples were taken at 1.3–1.8 m (silt), 3.6–4.1 m (silt), 4.2–4.7 m (silt), 7–7.2 m (fine sand). The ages mainly correspond to the Late Valdai (13–16 ka), with the upper early Holocene one (10.7 ka). The sedimentological characteristics indicate that these sediments are of aeolian origin.
The last site is in the Plyos incision valley itself. Although it has been suggested that both Upper Volga incision valleys do not contain terraces (Kvasov, 1979), we identified at least one apparent terrace in the Plyos incision valley (fig. 4). It is a sand body leaning towards the left side of the valley, across from the town of Plyos, where a section naturally created by a slope-wash processes was studied. It contains a 15-m thick sequence of alternating coarse, medium, and fine sands with pebbles and gravel displaying horizontal and cross lamination. We can confidently identify these deposits as alluvial sediments of the Volga River. We took 6 samples from this section for dating: two from in the upper, two from the middle, and two from the lower part of the sand body. OSL dating revealed these to be late MIS 6 – MIS 5 sediments. Ages are not entirely stratigraphically consistent, but such scatter is considered typical for quartz. All quartz ages are in the range 108–153 ka, and consistent with a single deposition period, except for one outlier – the sample with a quartz age of 59 ka. Since it is totally inconsistent with all the other ages, it is thought to be wrong and is not considered further.
3.3. GIA modeling. The reconstructions of GIA influence on the Upper Volga basin prove that the axis of the glacial forebulge affected the Rybinsk-Plyos part of the river. The axis for all time slices lies between Kostroma and Plyos. The width and height of the forebulge, the length and height of the “slope” to the glacier change over time. For 21 ka, the maximum relative elevation of the forebulge is 17 m, and the maximum lowering near the Volga River source is –77 m. However, we must note that during this time, the source was covered by the glacier (Astakhov et al., 2016). For 17.5 ka, the maximum elevation of the forebulge is 12 m, and the maximum lowering near the source is –85 m. For 15 ka, the maximum elevation of the forebulge is 9 m, and the maximum lowering near the source is –65 m. GIA modelling also allowed us to study the possible changes in the Volga’s profile throughout the last deglaciation that were caused by the glacial influence. The comparison of the modelled profiles with the modern one (fig. 5) shows no sufficiently strong GIA influence to skew the river profile completely towards the glacier – it mostly retains its shape. Still, GIA had a certain effect on the Upper Volga basin. Some skewing can be seen in the upmost reaches of the river, but it does not affect our key region. We can also see that during the LGM and deglaciation the profile was slightly elevated in the area close to the forebulge axis (Kostroma-Plyos, fig. 1). According to our reconstructions, the height of the glacial forebulge gradually decreased throughout the deglaciation, and the riverbed followed these changes.
Fig. 5.
Comparison of the present-day Upper Volga River profile with the ones built for 21, 17.5 and 15 ka using GIA modelling (Spada, Melini, 2019; Utkina, 2020).
Рис. 5. Продольные профили верхней Волги, построенные на временные срезы 21, 17.5 и 15 тыс. л. н. по палео-ЦМР, реконструированным с помощью программы SELEN4, моделирующей палеотопографию с учетом гляциоизостатических деформаций (Spada, Melini, 2019; Utkina, 2020).
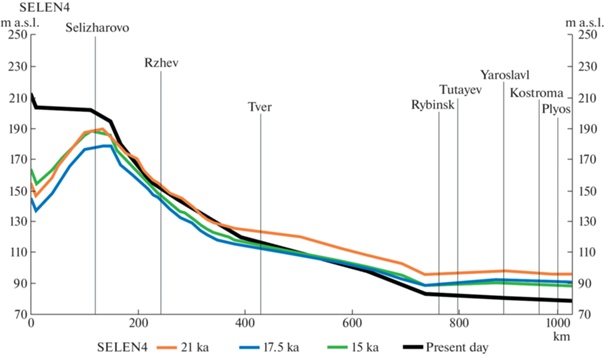
4. DISCUSSION
The most widely accepted Upper Volga evolution model (Kvasov, 1979) proposes that the Upper Volga River valley was formed after the MIS 2 proglacial lakes drained to the south. Both Upper Volga incision valleys formed during the lake drainage, but it was the formation of Plyos incision valley specifically that gave way to the south drainage and the river valley emergence. Following this model, we expected the landforms in the incision valleys to date back mostly to MIS 2 or shortly thereafter. Moreover, according to previous studies, there was supposed to be no alluvial sediments and river terraces in the incision valleys itself. Nevertheless, our field studies allowed us to locate these in the Plyos incision valley. The sand body we discovered there is confidently identified as an alluvial sediment and dates back to the late MIS 6 – MIS 5 time. Thus, we can refute the previously stated statement about the absence of terraces in the Plyos incision valley, and, in addition, determine the time when the valley should have already existed. If it is filled with the late MIS 6 – MIS 5 sediments, we can safely assume that the valley was formed long before MIS 2.
During the study of the Tutayev incision valley, the first terrace of the Volga River with a height of 8 m above the summer water level (91 m a.s.l.) was discovered. According to the OSL dating results, the terrace formation continued throughout the Holocene (from approx. 10 ka to approx. 1 ka). Other boreholes further up the slope did not reveal confidently identified alluvial or lacustrine deposits. The upper part of the valley slope is covered by slope deposits, and in the middle part a layer of presumably fluvioglacial deposits (dating back to 200–300 ka) was discovered overlying the till. 30 km upstream from Plyos a similar sediment was found being even older than the one near Tutayev (400–500 ka). Other recent studies of the Upper Volga valley sediments showed similar results in some other places in the valley (Utkina et al., 2022). For now, we are uncertain of the correct interpretation of these data, and further studies are required to resolve this issue. Another borehole from the same site allowed us to determine the Late Valdai–early Holocene aeolian sedimentation in the valley.
Following the GIA modelling results, we can assume that the proglacial lakes formation under the GIA influence can be ruled out for the Rybinsk-Plyos section of the Volga, since we can see no sufficiently strong GIA influence to skew the river profile completely towards the glacier. Moreover, we did not find actual proof of late Pleistocene lake deposits in the Upper Volga valley. Slight skew in the upper reaches near the Volga source might mean that the proglacial lakes existed there during Late Valdai, but there is no evidence for it happening to the Rybinsk-Plyos part of the river. However, the GIA effect appears to have influenced this part in some other way – the gradual decrease of the glacial forebulge throughout the deglaciation caused notable change in elevation of the Volga riverbed. This process should have caused an increase in the slope of the riverbed and, in turn, its postglacial cutting episode.
Thus, according to the data we have obtained on the geological and geomorphological structure of the incision valleys, their formation should have occurred in the Late Moscow (Late MIS 6) time, which is most convincingly evidenced by the Plyos incision valley terrace. Excluding the possibility of proglacial lakes emergence in the Upper Volga basin, the most likely mechanism for the incision valleys formation is the meltwater erosion caused by the meltwater flows of the Moscow (MIS 6) glaciation, when the glacier border was last the closest to the key area. If so, the Upper Volga valley was formed and became part of the larger Volga River system at late MIS 6. The GIA effect of the MIS 2 glacier was manifested through the forebulge evolution during the last deglaciation. The gradual decrease in the forebulge height caused the cutting episode which is confirmed by the Holocene terrace.
5. CONCLUSION
New data on the structure of the incision valleys and the possible GIA influence on the development of the Upper Volga valley in the Late Pleistocene allows us to clarify some available information about the Upper Volga Valley and its evolution. According to the most popular model of the Upper Volga emergence, its valley did not exist yet in MIS 2 and its basin was occupied by a proglacial lake system dammed by the MIS 2 glacier. The valley was formed around 14.5 ka, when the Plyos incision valley emerged, and the lake system was drained to the south, although same parts of the modern valley, like the Tutayev incision valley, were formed even prior to this event. Since other researchers also connected the formation of the incision valleys to the formation of the entire Upper Volga valley, we chose them as key regions in this study. Other than that, we considered possible GIA influence on the basin which might have served as one of the possible reasons for the proglacial lakes’ existence: the MIS 2 glacial forebulge cutting across the Rybinsk-Plyos river part could have skewed the topography of the basin towards the glacier, thus damming the waterflow between itself and the ice sheet.
It was found that most likely both incision valleys were formed in the MIS 6 (Late Moscow time) during the formation of a glacial meltwater runoff system connected to the retreat of the MIS 6 glacier. The late MIS 6 – MIS 5 terrace found in the Plyos incision valley serves as strong evidence of the valley existence during that time. No MIS 2 limnic or alluvial sediments were found in the valley, and the GIA modelling did not show any proof of possible lake formation due to the skew towards the glacier. During late MIS 2, the Upper Volga could have experienced a cutting episode caused by a gradual riverbed slope decrease, which occurred because of the glacial forebulge surface lowering as the ice sheet retreated. The accumulation began in the valley in the early Holocene and resulted in the formation of a terrace. This Holocene terrace was located in the Tutaev incision valley. Thus, the general configuration of the Upper Volga valley and its basin was established in the late MIS 6, when the incision valleys were also formed.
Список литературы отсутствует.
Дополнительные материалы отсутствуют.
Инструменты
Геоморфология и палеогеография