Микология и фитопатология, 2019, T. 53, № 4, стр. 197-209
The accumulation of heavy metals by macromycetes in Brest Region of the Republic of Belarus
T. N. Myslyva 1, *, N. V. Mikhalchuk 2, **, Yu. A. Bilyavskiy 3, ***, P. P. Nadtochiy 3, *
1 Belarus State Agricultural Academy
213407 Gorki, Republic of Belarus
2 Polesye Agrarian Ecological Institute of the National Academy of Sciences of Belarus
224020 Brest, Republic of Belarus
3 Zhytomyr National Agroecological University
10008 Zhytomyr, Ukraine
* E-mail: byrty41@yahoo.com
** E-mail: info@paei.by
*** E-mail: yura.bel.70@mail.ru
* E-mail: byrty41@yahoo.com
Поступила в редакцию 6.11.2017
После доработки 15.12.2018
Принята к публикации 21.12.2018
Аннотация
A research team has analysed heavy metals accumulation by various species of wild edible macromycetes, which grow within the boundaries of contaminated and conditionally background territories of natural forest ecosystems of Brest region of the Republic of Belarus. It has been found that within the boundaries of the studied territory, the concentration of heavy metals in fruit bodies of macromycetes is more species-specific and environment dependent than the nature of the substrate and the place of growth. Mycological products, collected within both technogenous polluted and conditionally background territories of Brest region are contaminated with heavy metals, which form the following ranked series in terms of the content of macromycetes in fruit bodies: Zn > Cu > Cd > Pb > Ni > Co. Consumption of mycological products with the following levels of contamination: Cd – 8.4–16.2 MAC; Zn – 6.5–11.0 MАC; Cu – 2.5–5.2 MAC; Pb – 8.4–16.2 MAC; Ni and Co – 1.5 MAC throughout life can lead to the increase in carcinogenic and non-carcinogenic health risks. The level of individual carcinogenic risk from consuming macromycetes contaminated with lead and cadmium is 0.1–3.4 × 10–2. Such a risk according to the international criterion scale is assessed as high, not acceptable for production conditions and for the population, and requires measures to be taken for its elimination or reduction.
INTRODUCTION
In the Republic of Belarus, mushroom produce is traditionally consumed by a large part of the population mostly due to its easy accessibility and low prices. Favourable natural conditions and a high level of afforestation stipulate the fact that the average annual mushroom biological and exploration reserves amount to 59.2 thousand tons and operational to 26.6 thousand tons respectively (Baginskiy, 2007; Koshedub, 2017). In addition, edible macromycetes prove to be a balanced natural complex of biologically active substances, essential amino acids and unsaturated omega-3 fatty acids. They also contain more than 20 macro- and microelements and A, B, E, D, PP vitamins (Yashchenko, 2012; Myslyva, Bilyavskiy, 2016).
However, under the unfavourable ecological conditions, due to the ever-growing anthropogenic impact on the environment, the mushroom produce can be heavily contaminated and damaging for the health of the population, since the mycobiota has a strong capacity for accumulating pollutants, and heavy metals in particular. Even 31 years after the Chernobyl disaster, the contamination of wild mushrooms with Cs137 in Belarus remains at a high level. According to the State Agency for the Protection and Monitoring of Forests “Bellesozashchita” the average content of this radionuclide in the fruit bodies of mushrooms growing in Brest region exceeds 1600 Bq/kg.
A review of available literature revealed that in Belarus there are practically no studies based on a long-term systematic research of the heavy metals accumulation in mushrooms and hazard assessment for humans consuming contaminated mycological products. However, the determination of heavy metal concentration in the fruiting bodies of mushrooms is essential in dietary intake studies: this acute problem is widely highlighted in scientific literature in many other countries (Wasser et al., 2012).
The purpose of the research was to study the peculiarities of Zn, Cu, Mn, Pb, Cd, Ni and Co accumulation in certain species of wild edible macromycetes with a tubular and lamellar hymenophore, which grow within the boundaries of contaminated and conditionally background territories of natural forest ecosystems of Brest Region of the Republic of Belarus, and to determine the carcinogenic and non-carcinogenic risk for the health of the population as a result of consuming contaminated mushroom products.
The investigation objectives are: 1) to determine the level of contamination of the topsoil, forest conditionally with heavy metals within the boundaries of natural forest ecosystems of contaminated and background territories; 2) to assess the degree of contamination and to find out the peculiarities of heavy metals accumulation in fruit bodies of certain edible mushroom species with either tubular or lamellar hymenophore; 3) to determine the degree of carcinogenic and non-carcinogenic risk for the health of the population from chemical substances which get into the bodies as a result of consuming contaminated macromycetes.
MATERIALS AND METHODS
The studied area and the object of research. The investigations were conducted in 2014–2016 in Brest and Kamenets districts of Brest Region of the Republic of Belarus. The research sites included forests adjoining Brest-Moscow M-1/E-30 highway exposed to the intensive anthropogenic impact (Brest region), as well as the forests within the boundaries of Belovezhskaya Pushcha National Park (Kamenets district), which belong to non-contaminated conditionally background territories (Figure 1).
The forests of the studied area is represented by pine, birch and oak plantations. The sod-podzolics (without subdivision) soils are predominant in the soil mantle of the contaminated and background territories (Umbric Retisols, WRB, 2014) (WRB, 2014).
The objects of the research were edible mushrooms of six species: Leccinum aurantiacum (Bull.) Gray, L. scabrum (Bull.) Gray, Suillus granulatus (L.) Roussel, S. variegatus (Sw.) Richon, Cantharellus cibarius Fr., Russula xerampelina (Schaeff.) Fr.
Sampling and analysis of soil and forest duff. The soil samples were taken from July to September in accordance with the requirements of ISO 10381-4:2003, IDT. They were selected from the sites of 50 × 200 m. One combined soil sample consisted of 8 to 10 point samples. Two types of soil samples were selected (taken): the upper organic-mineral horizon (forest duff) 0–3 см and mixed sample of the upper soil horizons 0–15 см. The acid soluble (1N HCl extractant) and exchange (extractant – buffered ammonium and acetate extract from pH 4.8) forms of Zn, Cu, Mn, Pb, Cd, Ni and Co in soil samples and forest conditionally were determined by the method of atomic and absorption spectrometry on SOLAAR MkII-M6 Double Beam AAS device.
Selection and analysis of mushrooms. Both the soil and forest conditionally samples and the mycological produce were collected from the same sites from July to September. Every mixed sample contained at least 3 fruit bodies. In total, 63 mixed samples of mushrooms were selected and analysed, including carpophore, hymenophore and stipes. All collected fruit bodies of macromycetes were mechanically cleansed from dirt with a plastic knife, rinsed with distilled water, cut into pieces and dried in the oven at the temperature of 65°C reaching air-dry state. Then the samples were reduced to fragments in the laboratory mill and sifted through a sieve with 1 mm diameter mashes. The residues on the sieve were ground in the agate mortar, then added to the sieved part and thoroughly mixed. The mineralization of mushroom samples was done through dry ashing in accordance with the standard 26929-94. Acidic extraction of heavy metals from the ash was carried out with a dilute 1 : 1 HNO3. The concentration of heavy metals was determined by the atomic absorption spectrometer with a flame atomizer SOLAAR MkII-M6 Double Beam AAS in accordance with the standard 30178-96.
Features of accumulation of heavy metals. The biological absorption coefficient (BAC) was used to analyse the ability of the species of macromycetes to accumulate chemical elements. It was calculated as the ratio of the content of the element in the fruit bodies of mushrooms to its background content in the soils of Brest region (Matveev, Bordon, 2013).
The indicator of element biotics (IEB) (Glazovskiy, 2006) was used to estimate the biogeochemical connection between the chemical composition of a living organism and the biosphere. It was calculated as the ratio of the content of the element in fruit bodies of mushrooms to its clark in the lithosphere (for Belarus) (Matveev, Bordon, 2013).
The accumulation coefficient (AC) was used to characterize the peculiarities of the transition of chemical elements from substrate to fungi. It was calculated as the ratio of the content of the element in fruit bodies of mushrooms to the concentration of exchange and acid-soluble forms of chemical elements in the forest conditionally (Ivanov et аl., 2008).
The hazard ratio of the heavy metals content (Chaz) was used to assess the level of contamination of macromycetes. It was calculated as the ratio of the average content of the element in the fruit body to the value of its maximum allowed concentration.
Assessment of non-carcinogenic and carcinogenic risk. The assessment of the degree of non-carcinogenic and carcinogenic risk was carried out according to conventional techniques (Onishchenko et al., 2002; R 2.1.10.1920-04, 2004). The value of the hazard quotient of the heavy metals content (НQ) in fruit bodies of separate species of macromycetes was determined for each element investigated as a quotient from dividing the daily intake of the element into its reference dose. The total hazard index (HI) was determined as the sum of hazard indices of each separate heavy metal. The assessment of the carcinogenic risk was made by calculating the individual and total risk. The individual carcinogenic risk was determined as the multiplication of the daily intake of the element in the body to the factor of incidence of carcinogen, whereas the total risk was determined as the sum of individual risks identified for each carcinogens. When determining the risk, we considered the fact that the season of wild growing mushrooms consumption lasts 6 months (from May to October). The daily consumption of mushrooms amounts to 0.143 kg of fresh weight for adults and adolescents over 14 years old and 0.071 kg – for children and adolescents under 14 years old (EFSA Journal, 2012). The average body weight of the representatives of various age groups – children at the age of 10–14 years, adolescents of 14–18 and adults – amounted to 43 kg, 61 kg and 70 kg respectively (Zsigmond et al., 2015).
Microsoft Excel 2013 and Statistica 12.0 applied program package were used to process experimental data.
RESULTS AND DISCUSSION
Heavy metals in soil and forest conditionally. The general specific feature of the investigated territory is that the content of both exchange and acid soluble forms of Zn, Сu, Mn, Ni and Со is higher in the forest duff than in 0–15 cm soil layer, which is a common feature of all soils in the temperate zone forests (Table 1).
Table 1.
The heavy metals content in forest duff (depth 0–3 cm) and mixed sample of the upper soil horizons (depth 0–15 cm) in forest ecosystems on the territory of Brest Region of the Republic of Belarus
Sampling depth and number of samples | Content of element, мg/kg air-dry mass | ||||||
---|---|---|---|---|---|---|---|
Zn | Cu | Mn | Pb | Cd | Ni | Co | |
Technogenous polluted territory | |||||||
Forest duff, 0–3 cm, n = 12 рН = 3.6–3.8 | $\frac{{12 \pm 0.6}}{{17 \pm 0.9}}$ | $\frac{{1.3 \pm 0.1}}{{1.5 \pm 0.1}}$ | $\frac{{89 \pm 5}}{{203 \pm 11}}$ | $\frac{{1.3 \pm 0.1}}{{3.7 \pm 0.2}}$ | $\frac{{0.11 \pm 0.01}}{{0.14 \pm 0.01}}$ | $\frac{{0.10 \pm 0.01}}{{0.35 \pm 0.2}}$ | $\frac{{0.11 \pm 0.05}}{{0.48 \pm 0.3}}$ |
Umbric Retisols, 0–15 cm, n = 12 рН = 4.9–5.1 | $\frac{{0.42 \pm 0.1}}{{0.89 \pm 0.04}}$ | $\frac{{0.63 \pm 0.05}}{{1.12 \pm 0.1}}$ | $\frac{{15 \pm 0.8}}{{35 \pm 2}}$ | $\frac{{3.17 \pm 0.2}}{{6.33 \pm 0.3}}$ | $\frac{{0.5 \pm 0.03}}{{0.6 \pm 0.03}}$ | $\frac{{0.05 \pm 0.03}}{{0.14 \pm 0.08}}$ | $\frac{{0.05 \pm 0.03}}{{0.32 \pm 0.2}}$ |
Conditionally background territory | |||||||
Forest duff, 0–3 cm, n = 9 рН = 3.8–4.0 | $\frac{{73 \pm 4}}{{104 \pm 5}}$ | $\frac{{9.5 \pm 0.5}}{{17 \pm 0.9}}$ | $\frac{{120 \pm 7}}{{273 \pm 15}}$ | $\frac{{3.1 \pm 0.2}}{{8.9 \pm 0.5}}$ | $\frac{{0.5 \pm 0.03}}{{0.7 \pm 0.04}}$ | $\frac{{1.1 \pm 0.05}}{{3.8 \pm 0.2}}$ | $\frac{{0.53 \pm 0.03}}{{1.5 \pm 0.08}}$ |
Umbric Retisols, 0–15 cm, n = 9 рН = 5.1–5.3 | $\frac{{5.7 \pm 0.3}}{{8.0 \pm 0.434}}$ | $\frac{{0.14 \pm 0.01}}{{1.3 \pm 0.1}}$ | $\frac{{109 \pm 6}}{{249 \pm 13}}$ | $\frac{{1.5 \pm 0.1}}{{4.2 \pm 0.3}}$ | $\frac{{0.6 \pm 0.03}}{{0.8 \pm 0.04}}$ | $\frac{{0.10 \pm 0.01}}{{0.34 \pm 0.02}}$ | $\frac{{0.15 \pm 0.01}}{{0.71 \pm 0.04}}$ |
МАС (GN 2.1.7.12-1-2004; GN 1.7.2041-06) | 23.0 | 3.0 | 80.0
(acid soluble forms) 400.0 (exchange forms) |
6.0 | 0.5 | 4.0 | 5.0 |
Clark (Matveev, Bordon, 2013) | 36.6 | 16.2 | 369.3 | 14.9 | 3.6 | 11.9 | 7.8 |
It is necessary to note, that the main sources of soil pollution with heavy metals in Brest region are emissions from vehicles, energy facilities and industrial enterprises (Kakareka, Kuharchik, 2012). Besides, special geographical location of Belarus introduced a transboundary component in atmospheric deposition on its territory (Mikhalchuk, 2017). In particular, the amount of lead participating in a transboundary transfer and falling into the territory of Belarus, only from the closest developed western countries (Poland, Germany) and Russia can be at least 355 tons per year, cadmium – more than 15 tons per year (Tonchevski, 2015).
The forest duff within the boundaries of the conditionally background territory proved to be more contaminated with such elements as Zn (3.18 МРС) and Сu (3.08 МРС), whereas the content of Mn exceeded the maximum allowed concentration both in the soil and in the forest duff by 1.8 and 2.0 times respectively. The forest duff within the boundaries of the technogenous-contaminated areas also contained manganese in quantities that on average exceeded the threshold concentration by 1.5 times. The above results are connected not only with the effects of the technogenic factors, but mainly with the biophilic factors, they are active in the biological cycle and accumulate quite intensively in the forest topsoil. A comparatively high content of manganese is the result of its biological accumulation in conditions of a very long interaction of forest vegetation with the soil, which is very typical of the indigenous forests of Belovezhskaya Pushcha (Mikhalchuk et al., 2016).
Besides, it is common knowledge that woody vegetation participates actively in the podzol paedogenesis formation and in the redistribution of nutrients and microelements along the soil profile. In particular, oak leaves have considerable concentrations of manganese (ВАС = 4.71), fresh forest duff and young needle litter accumulate significant amount of copper (ВАС = = 3.35–5.97), Betula pendula (L.) Roth has considerable concentrations of zinc, its accumulation coefficient in leaves and young branches amounts to 5–7 units. It is this fact that explains why the content of Zn, Сu and Mn in the forest conditionally within the conditionally background territory, which is presented by pine, oak and birch stand exceeds the content of these elements in the forest conditionally on technologically contaminated territory (Myslyva, Bilyavskiy, 2016).
The content of exchange and acid soluble forms of lead in the soil cover of the contaminated area is higher than in the forest duff. A high content of lead in the soil in the investigated area proved to be of the technogenic nature, and it is predetermined, in the first place, by the lateral intake of contaminated aerial masses by forest ecosystems and flood runoff from motor transport landscapes bordering on these ecosystems or just crossing them. Similar regularities were also found in the investigations carried out by Ukrainian scientists (Myslyva, 2013). The source of cadmium emission in the soil within the polluted and conditionally background areas exceeds maximum allowable concentration on average by 1.2 times and the index of background content for Brest region by 5–6 times. It is the leaf and needle litters, which absorb this pollutant from the contaminated air masses coming from nearby transport landscapes.
Features of the accumulation of heavy metals by macromycetes. The concentration of chemical substances in the fruit bodies of macromycetes is species and environment-specific (Sazanova et al., 2017), and also depends on the content of chemical elements in parent species, their mineral composition, soil type, its agrochemical, physical and chemical properties as well as on the chemical composition of forest conditionally (Myslyva, Bilyavskiy, 2016). The average content of chemical elements in the studied species of mushrooms was correlated with the data obtained by other researchers, in particular, carried out in similar soil and climatic conditions of Poland. For the contaminated areas, the average zinc content in fruit bodies of macromycetes was 172–270 mg/kg; copper – 26–95; manganese – 5–85; lead – 0.5–2.0; cadmium – 0.2–2.0; nickel – 0.2–1.6; cobalt – 0.4–1.5 mg/kg of dry weight. For macromycetes growing within the conditionally background territories, the average zinc content in the fruit bodies was 75–224 mg/kg; copper – 7–44; manganese – 4–31; lead – 0.1–1.1; cadmium – 0.5–3.0; nickel – 0.3–1.0; cobalt – 0.3–0.5 mg/kg of dry weight (Table 2).
Table 2.
The content of heavy metals in the fruit bodies of macromycetes, growing within the technogenous polluted territory (PT) and conditionally background territory (BТ) of Brest Region of the Republic of Belarus and neighboring countries
Mushroom species | Content of elements, mg/kg air-dry mass | Source | ||||||
---|---|---|---|---|---|---|---|---|
Zn | Cu | Mn | Pb | Cd | Ni | Со | ||
Cantharelluscibarius (PТ) | 263 ± 47 | 95 ± 19 | 85 ± 19 | 0.81 ± 0.28 | 0.46 ± 0.12 | 1.60 ± 0.24 | 1.52 ± 0.29 | Authors’ data |
C. cibarius | 13.2–20.4 | 13.2–15.3 | – | 0.04–13.2 | 0.036–0.19 | 0.1–2.64 | 13.2–20.4 | Sazanova et al. (2017): Finland, Poland |
95.2–139 | 13.0–28.4 | – | 43.7–49.8 | 2.24–3.37 | – | – | Záhorcová et al. (2016): Slovakia | |
69–100 | 33–77 | 20–63 | – | 0.49–0.67 | – | – | Brzezicha-Cirocka et al. (2016): Eastern Poland | |
34.05–69.15 | 20.69–45.92 | 16.1–19.5 | – | 0.17–0.5 | 0.33–0.79 | 0.12 | Zsigmond et al. (2015): Romania, urban region | |
– | 34–133 | 4.3–55.0 | 0.16–1.0 | 0.038–0.11 | 1.5–3.4 | 0.004–0.26 | Koroleva, Ohrimenko (2015): Kaliningrad Region, Russia | |
– | – | – | 1.71 | 0.44 | 2.08 | – | Pelkonen et al. (2006): Finland | |
140.6 | – | – | – | – | – | – | Georgescu, Busuioc (2011): Dambovita county, Romania | |
57.5 | 32.7 | – | 1.63 | 1.0 | 0.83 | – | Stankeviciene D (2004): Lithuania | |
Suillusgranulatus (PТ) | 189 ± 33 | 30 ± 5 | 7 ± 1.5 | 0.57 ± 0.14 | 0.24 ± 0.06 | 0.19 ± 0.03 | 0.51 ± 0.08 | Authors’ data |
– | 37.4–60.6 | – | – | – | – | 0.06–1.02 | Svoboda, Chrastny (2007): rural area, Czech Republic | |
Suillus species | 32.8–128.8 | 0.06–28.2 | 27.6–71.7 | 0.03–27.7 | 0.15–8.95 | 0.94–18.3 | – | Sazanova et al. (2017): Eastern Poland |
S. luteus | 78–180 | 11–33 | 24–62 | – | 0.19–0.80 | – | – | Brzezicha-Cirocka et al. (2016): Eastern Poland |
44.55 | 26.33 | – | 0.78 | 2.98 | 1.04 | 0.28 | Mleczek et al. (2013): Poland | |
S. variegatus (ВТ) | 75 ± 16 | 7 ± 1.5 | 4 ± 0.8 | 2.13 ± 0.48 | 1.16 ± 0.24 | 0.26 ± 0.07 | 0.45 ± 0.07 | Authors’ data |
S. variegatus | 90 | 19 | 13 | 0.2 | 1.0 | – | 0.07 | Szubstarska et al. (2012): Northern Poland |
– | – | – | 1.11 | 1.46 | 1.17 | – | Pelkonen et al. (2006): Finland | |
70.5–73.3 | – | – | – | – | – | 0.15–0.22 | Borovička, Řanda (2007): Czech Republic | |
Leccinum scabrum (PТ) | 173 ± 35 | 26 ± 5 | 11 ± 2 | 0.53 ± 0.12 | 1.99 ± 0.45 | 0.62 ± 0.16 | 0.42 ± 0.07 | Authors’ data |
L. scabrum (ВТ) | 224 ± 63 | 25 ± 4 | 16 ± 3 | 0.11 ± 0.02 | 3.24 ± 0.68 | 0.95 ± 0.17 | 0.31 ± 0.05 | |
L. scabrum | – | – | – | 0.4 | 0.8 | – | – | Sazanova et al. (2017): Eastern Poland |
140–260 | 14–39 | 6.4–43.0 | 0.78–7.5 | 1.2–8.8 | 0.76–0.91 | 0.011–0.48 | Zhang et al. (2013): Sudety Mountains, Poland | |
– | 22.0–29.6 | – | – | – | – | 0.09–0.15 | Svoboda, Chrastny (2007): rural region, Czech Republic | |
15.83–68.33 | 7.1–15.77 | – | 0.1–15.4 | 0.33–2.60 | – | Stankeviciene (2004): Lithuania | ||
L. aurantiacum (PТ) | 271 ± 52 | 60 ± 14 | 5 ± 0.8 | 1.92 ± 0.28 | 0.68 ± 0.15 | 0.51 ± 0.07 | 0.54 ± 0.08 | Authors’ data |
L. aurantiacum | 110.37 | – | – | 0.38–33.8 | 0.35–2.36 | 8.51 | – | Sazanova et al. (2017): Eastern Poland |
20–320 | 11–150 | 5.4–73.0 | – | – | – | – | Brzezicha-Cirocka et al. (2016): Eastern Poland | |
72.04–162.42 | 12.65–22.89 | – | 0.82–1.42 | 1.96–3.94 | 1.92–2.99 | 0.64–1.48 | Mleczek et al. (2013b): Poland | |
Russula xerampelina (ВТ) | 95 ± 15 | 44 ± 11 | 31 ± 9 | 1.14 ± 0.27 | 0.47 ± 0.09 | 0.32 ± 0.04 | 0.37 ± 0.06 | Authors’ data |
R. xerampelina | – | 43–79 | 14–15 | 0.07–1.3 | 1.2–4.7 | 4.2–5.0 | 0.009–0.03 | Koroleva, Ohrimenko (2015): Kaliningrad Region, Russia |
19.7–99.6 | – | – | – | – | – | – | Georgescu, Busuioc (2011): Dambovita county, Romania | |
Russula species | 19.3–58.2 | 10.8–73.0 | 24.2–26.4 | 0.77–2.6 | 0.06–2.0 | 3.2 | – | Sazanova et al. (2017): Eastern Poland |
30.4–146.7 | 6.6–34.1 | 11.1–219.2 | – | – | 0.3–2.1 | 0.009 | Elekes, Busuioc (2013): Targoviste, Romania | |
– | 25.2–64.0 | – | – | – | – | 0.29–1.81 | Svoboda, Chrastny (2007): rural area, Czech Republic |
Mushrooms can accumulate heavy metals even when their concentration in the soil is relatively low (Falandysz et al., 2003; Kula, 2011; Sazanova et al., 2017). Under the conditions of forest ecosystems subject to the intensive technogenic effects (highwayside forest stock) and irrespective of macromycetes species their fruit bodies accumulate copper (ACBАА = 20.73–76.37; ACА = 16.91–62.3) and zinc (ACBАА = 16.04–23.02; ACА = 11.29–16.20) most intensively (Table 3). The maximum zinc accumulating capacity has shown Leccinum aurantiacum. High capacity of this species to accumulate high concentrations of Zn and Cu has also been revealed in investigations carried out in conditions of Ukrainian Polesie (Bilyavskiy et al., 2016), within the boundaries of Yaroslavl Region (Pleshcheevo Lake National Park) (Pelgunov, Pelgunova, 2015) and within the boundaries of Poland (Mleczek et al., 2013b). The highest ability to concentrate both exchange and acid-soluble forms of copper was demonstrated by Cantharellus cibarius. Other researchers (Solomko et al., 1986; Alonso et al., 2003) also registered the ability of this species to accumulate Cu.
Table 3.
Coefficients of accumulation of heavy metals in the fruit bodies of macromycetes
Species | Element | ACBАА | ACA | BAC | IEB |
---|---|---|---|---|---|
Technogenous polluted territory | |||||
Leccinum scabrum | Zn | 14.66 | 10.31 | 12.97 | 4.71 |
Cu | 20.73 | 16.91 | 11.18 | 1.59 | |
Mn | 0.12 | 0.05 | 0.18 | 0.03 | |
Pb | 0.42 | 0.14 | 0.13 | 0.04 | |
Cd | 18.09 | 14.21 | 19.90 | 0.55 | |
Ni | 6.20 | 1.77 | 0.26 | 0.05 | |
Co | 3.82 | 0.88 | 0.20 | 0.05 | |
L. aurantiacum | Zn | 23.02 | 16.20 | 20.38 | 7.40 |
Cu | 48.39 | 39.47 | 26.09 | 3.70 | |
Mn | 0.05 | 0.02 | 0.08 | 0.01 | |
Pb | 1.51 | 0.52 | 0.47 | 0.13 | |
Cd | 6.18 | 4.86 | 6.80 | 0.19 | |
Ni | 5.00 | 1.43 | 0.21 | 0.04 | |
Co | 4.91 | 1.13 | 0.26 | 0.07 | |
Cantharellus cibarius | Zn | 22.38 | 15.74 | 19.80 | 7.20 |
Cu | 76.37 | 62.30 | 41.17 | 5.85 | |
Mn | 0.95 | 0.42 | 1.42 | 0.23 | |
Pb | 0.64 | 0.22 | 0.20 | 0.05 | |
Cd | 4.18 | 3.29 | 4.60 | 0.13 | |
Ni | 16.00 | 4.57 | 0.67 | 0.13 | |
Co | 13.64 | 3.13 | 0.71 | 0.19 | |
Suillus granulatus | Zn | 16.04 | 11.29 | 14.20 | 5.16 |
Cu | 23.81 | 19.42 | 12.83 | 1.82 | |
Mn | 0.08 | 0.03 | 0.11 | 0.02 | |
Pb | 0.45 | 0.15 | 0.14 | 0.04 | |
Cd | 2.18 | 1.71 | 2.40 | 0.07 | |
Ni | 1.90 | 1.36 | 0.08 | 0.02 | |
Co | 4.55 | 1.56 | 0.24 | 0.06 | |
Conditionally background territory | |||||
Leccinum scabrum | Zn | 3.07 | 2.16 | 16.85 | 6.12 |
Cu | 2.65 | 1.43 | 10.65 | 1.51 | |
Mn | 0.14 | 0.06 | 0.27 | 0.04 | |
Pb | 0.03 | 0.01 | 0.02 | 0.01 | |
Cd | 6.48 | 4.63 | 32.40 | 0.90 | |
Ni | 0.87 | 0.25 | 0.40 | 0.08 | |
Co | 0.58 | 0.21 | 0.15 | 0.04 | |
Suillus variegatus | Zn | 1.02 | 0.72 | 5.62 | 2.04 |
Cu | 0.76 | 0.41 | 3.04 | 0.43 | |
Mn | 0.04 | 0.02 | 0.07 | 0.01 | |
Pb | 0.65 | 0.22 | 0.49 | 0.13 | |
Cd | 2.32 | 1.66 | 11.60 | 0.32 | |
Ni | 0.24 | 0.07 | 0.11 | 0.02 | |
Co | 0.85 | 0.30 | 0.21 | 0.06 | |
Russula xerampelina | Zn | 1.30 | 0.92 | 7.16 | 2.60 |
Cu | 4.80 | 2.60 | 19.30 | 2.74 | |
Mn | 0.26 | 0.11 | 0.52 | 0.08 | |
Pb | 0.37 | 0.13 | 0.28 | 0.08 | |
Cd | 0.94 | 0.67 | 4.70 | 0.13 | |
Ni | 0.29 | 0.08 | 0.13 | 0.03 | |
Co | 0.70 | 0.25 | 0.18 | 0.05 |
Copper (ACBАА = 2.65–4.80; ACА = 1.43–2.60) and zinc (ACBАА = 1.02–3.07; ACА = 0.72–2.16) accumulated intensively in the fruit bodies of macromycetes growing within the limits of the conditionally background territory. A characteristic feature of zinc is its ability to bind with humic acids with conversion into less mobile compounds – zinc humates. Thus, the consolidation of Zn with sandy-loamy soils on the conditioned-background territory occurs, and a less intensive accumulation of it with the fruit bodies of macromycetes takes place. Copper is more mobile in the upper genetic horizons of the soil than zinc, and its maximum mobility occurs in forest conditionally with pH in the range of 3.6–3.8 pH units. For this reason, copper accumulates most intensively in mushrooms that grow within the National Park “Belovezhskaya Pushcha”. Leccinum scabrum is the absorber of zinc, and Russula xerampelina is the absorber of copper. A high ability to accumulate zinc was established for species of genus Leccinum in the studies by Mleczek et al. (2013b). The intensive accumulation of copper by R. xerampelina is obviously connected with the place where it grows, and with the ability of the given species to accumulate copper, which was highlighted by some scientists (Elekes, Busuioc, 2013; Bilyavskiy et al., 2016).
Every species of macromycetes investigated is characterized by low or extremely low ability to accumulate lead (ACBАА = 0.03–1.51; ACА = 0.01–0.52). These results also correlate with the results obtained in Finland, which, in particular, revealed a low capacity for lead accumulation by Leccinum species (Pelkonen et. al., 2006). A low capacity for lead concentration for Leccinum aurantiacum and Cantharellus cibarius was demonstrated in the studies carried out on the territory of the Republic of Poland (Mleczek et al., 2013b). The reason for a rather low intensity of lead accumulation by symbiotrophic macromycetes is that this element accumulates in the upper genetic horizons of the soil profile. These horizons are rich in organic matter that binds this element. Lead can be a part of the Fe and Mn oxides, which are abundant in the soil of the studied area, thus becoming inaccessible.
Manganese is the least accumulated element by mushrooms growing on both contaminated and conditionally background territories. Depending on the location and macromycetes species the coefficient of its biological absorption varies within the limits of 0.02 to 0.42 for acid soluble and from 0.04 to 0.95 – for exchange forms. Manganese is the element of weak accumulate and intake for 30% of the species investigated. The above findings correlate with the data obtained under the investigation into the peculiarities of manganese accumulation by macromycetes on Irtysh land (Sibirkina, 2009). C. cibarius accumulated manganese most intensively. The manganese accumulation coefficient for this species reached 0.95 for exchange and 0.42 for acid-soluble forms. The ability of this species to accumulate manganese is highlighted in other investigations (Sesli et al., 2008). Since the values of the manganese biological accumulation coefficients do not exceed meaning one, it can be assumed that it enters into the fruit bodies of macromycetes exclusively from the substrate. This statement is also true for the accumulation of lead.
Cadmium is most intensively accumulated by Leccinum scabrum (ACBАА = 18.09; ACА = 14.21) and L. aurantiacum (ACBАА = 6.18; ACА = 4.86), growing within the technogenous polluted territory. L. scabrum (ACBАА = 6.48; ACА = 4.63) and Suillus variegatus (ACBАА = 2.32; ACА = 1.66) also differed in their ability to accumulate this pollutant; growing within a conditionally background territory. The investigations of Ivanov et al. (2017) point out that the content of cadmium in fruit bodies of mushrooms increases 4–5 times even with insignificant soil contamination, which is due to high mobility of the given element, and our findings confirm it. The ability of Leccinum scabrum to accumulate increased amounts of cadmium is also underlined by other investigations which were carried out in conditions of Ukrainian Polesie (Bilyavskiy et al., 2016), Romania (Zsigmond et al., 2015), and Poland (Mleczek et al., 2013b). It is necessary to note that L. scabrum belongs to the Boletaceae family which representatives are predisposed to cadmium bioaccumulation. The ability of Boletus еdulis, B. badius and B. chrysenteron species to accumulate this pollutant was registered in some investigations (Pelkonen et al., 2006; Mleczek et al., 2013a, 2013b; Zsigmond et al., 2015; Koroleva, Ohrimenko, 2015; Bilyavskiy et al., 2016). The reason of active cadmium accumulation by macromycetes within the limits of conditionally background territory, in our opinion, is its increased mobility because of high values of the soil substrate pH, which amount to 3.8–4.0 pH units. A considerable geochemical affinity between Zn and Cd predetermines similar transfer of these metals to the plants and fruit bodies of macromycetes, which obviously absorb more mobile cadmium instead of zinc. Besides, in many biochemical processes cadmium can replace zinc, since their chemical properties are almost similar.
The content of nickel and cobalt in the substrate did not exceed the critical concentration, though these elements were accumulated in surplus quantities mainly in the fruit bodies of macromycetes growing within highwayside forests (ACBАА = 1.9–16.0; ACА = 1.36–4.57 for Ni and ACBАА = 3.82–13.64; ACА = 0.88–3.13 for Co). The nickel and cobalt accumulator was Cantharellus cibarius. The ability of species belonging to symbiotrophs, in particular, species of the genus Suillus to accumulate cobalt is also indicated by Zsigmond et al. (2015). In general, a higher concentration of Zn, Cu, Cd, Ni and Co in the fruit bodies of macromycetes was observed in comparison with the substrate on which they grow, whereas the content of lead and manganese was higher in the substrate.
Contamination of mushrooms with heavy metals. Despite the fact that soil, and forest floor within the limits of Belovezhskaya Pushcha National Park contain larger quantities of zinc and copper, than soil and forest bedding in the limits of the technogenous contaminated territory, the hazard coefficients of their content in macromycetes, which grow in the limits of conditionally background territory, have the lowest values as compared with such for the technogenous contaminated territory (Table. 4).
Although the soil in the environments of highway contained the quantities of zinc and copper equivalent to only 0.02 and 0.21 МАС respectively, the hazard coefficient of content of the above elements in the fruit bodies of macromycetes reached the values of 8.6–13.6 for zinc and 2.6–9.5 for copper. Within the limits of natural forest ecosystems, they were 3.7–11.2 for zinc and 0.7–4.4 for copper. This fact confirms that a species and ecological environment are the main factors, which influence the accumulation of heavy metals by macromycetes which was also noted by a number of researchers (Sibirkina, 2009; Koroleva, Ohrimenko, 2015; Sazanova et al., 2017; Ivanov et аl., 2017).
There is one more fact to consider. The contamination of the macromycetes fruit bodies growing within both contaminated and conditionally background territories with lead is relatively similar. The coefficient of hazard (Chaz) of lead content in the fruit bodies of macromycetes growing on the technogenous-disturbed territory varied from 1.1 to 3.8, whereas its value on the territory of natural forest ecosystems amounted to 0.2–4.0 on the average depending on the variety of macromycetes (Table 4).
Table 4.
Hazard coefficient of heavy metals (Chaz) in the fruit bodies of macromycetes
Species | Hazard coefficient of (Chaz) | |||||
---|---|---|---|---|---|---|
Zn | Cu | Pb | Cd | Ni | Co | |
Technogenous polluted territory | ||||||
Cantharellus cibarius | 13.17 | 9.47 | 1.62 | 4.60 | 3.20 | 3.00 |
Suillus granulatus | 9.44 | 2.95 | 1.14 | 2.40 | 0.38 | 1.00 |
Leccinum scabrum | 8.63 | 2.57 | 1.06 | 19.90 | 1.24 | 0.84 |
L. aurantiacum | 13.55 | 6.00 | 3.84 | 6.80 | 1.00 | 1.08 |
Average meaning | 11.20 | 5.25 | 1.92 | 8.43 | 1.46 | 1.48 |
Conditionally background territory | ||||||
Russula xerampelina | 4.76 | 4.44 | 2.28 | 4.70 | 0.64 | 0.74 |
Suillus variegatus | 3.74 | 0.70 | 4.00 | 11.60 | 0.52 | 0.90 |
Leccinum scabrum | 11.20 | 2.45 | 0.20 | 32.40 | 1.90 | 0.62 |
Average meaning | 6.57 | 2.53 | 2.16 | 16.23 | 1.02 | 0.75 |
MАC, mg/kg | 20 | 10 | 0.5 | 0.1 | 0.5 | 0.5 |
The similar tendency was observed with cadmium. Besides, it has been revealed that the fruit bodies of Leccinum scabrum growing on both contaminated and conditionally background territory had the maximum cadmium contamination equivalent – 19.9–32.4 of critical concentration, whereas the average content of the given pollutants in the soil did not exceed 1.0–1.2 of critical concentration. The maximum contamination with both nickel and cobalt was observed in the fruit bodies of Cantharellus cibarius, which shows the ability to accumulate the given pollutants, as it is highlighted in the research of Murati et al. (2015).
Carcinogenic and non-carcinogenic risk. The research has provided the opportunity to determine the risk of the human’s intake, including carcinogenic one, because of consuming such species of macromycetes contaminated with heavy metals, which were calculated for various age groups. The risks were assessed according to the value of hazard quotient (HQ) for every metal and the value of the total hazard index (HI) which is determined as a sum of all hazard quotients. If the total hazard quotient does not exceed meaning one (1), the probability of developing hazardous effects in humans at daily substance intake during the lifetime is inessential, and this effect is characterized as admissible. If the total hazard quotient exceeds meaning one (1), the probability of developing hazardous effects in human’s increases in proportion to the increase in HI. In macromycetes growing on both the technogenous contaminated and on the background territory the highest hazard quotient was revealed for cadmium (HQ = 0.10–1.32) and copper (HQ = 0.10–1.02) (Table 5).
Table 5.
The total hazard index (HI) of the heavy metals content in the fruit bodies of separate species of macromycetes
Species | Age-groups of the population living in the study region | ||
---|---|---|---|
10–14 years | 14–18 years | adults | |
Technogenous polluted territory | |||
Cantharellus cibarius | 1.28 | 1.82 | 1.59 |
Suillus granulatus | 0.48 | 0.68 | 0.59 |
Leccinum scabrum | 1.02 | 1.45 | 1.26 |
L. aurantiacum | 1.00 | 1.42 | 1.24 |
Conditionally background territory | |||
Russula xerampelina | 0.69 | 0.98 | 0.85 |
Suillus variegatus | 0.59 | 0.84 | 0.73 |
Leccinum scabrum | 1.44 | 2.05 | 1.78 |
In terms of the magnitude of non-carcinogenic risk among all species of macromycetes growing on the technogenous-contaminated territory, only Suillus granulatus proved to be the safest when consumed as a food product. This species along with Russula xerampelina growing within the background territory proved to be the safest as to the level of their contamination with heavy metals.
When assessing the total risk of damage to certain organs and systems of the human body, it has been established that when eating macromycetes that grow both within the contaminated area and on a conditionally background area, the most likely adverse effects can be for gastrointestinal tract and liver (HQcont = = 1.84–2.62; HQbackgr = 0.67–0.96), and hematopoietic organs (HQcont = 1.84–2.62; HQbackgr = 1.91–2.71) (Figs 2, 3). Moreover, the risk of negative effects on the hematopoietic organs when eating macromycetes grown on a conditionally background area is higher than for a contaminated area due to higher levels of macromycetes contamination by cadmium. The maximum contribution to the total risk is made by Cu, Ni and Cd. Regardless of the location of growth and the level of contamination of macromycetes, the maximum non-carcinogenic risk is established for the age group of adolescents aged 14–18.
Fig. 2.
The total risk of damage to certain organs and systems of the human body when eating macromycetes that grow within a technogenous polluted area, HQcont.
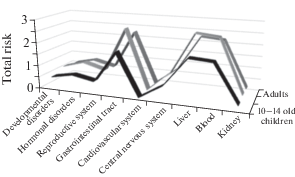
Fig. 3.
The total risk of damage to certain organs and systems of the human body when eating macromycetes that grow within a conditionally background territory, HQbackgr.
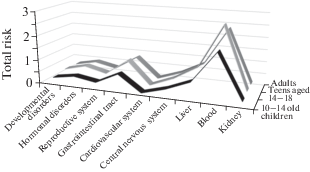
The assessment of carcinogenic risk, which is understood as the probability of the increase in the incidence of neoplasms in humans due to the peroral effects of chemical carcinogens, was done by calculating the values of individual, total and population risks. Studied. Lead and cadmium as substances with a proven carcinogenic effect, were selected among the chemicals (Table 6).
Table 6.
The total carcinogenic risk at peroral intake of heavy metals consuming polluted macromycetes
Species | Age-groups of the population living in the studied region | |
---|---|---|
10–14 years | 14–18 years and adults | |
Technogenous polluted territory | ||
Cantharellus cibarius | 0.03 | 0.05 |
Suillus granulatus | 0.01 | 0.03 |
Leccinum scabrum | 0.10 | 0.21 |
L. aurantiacum | 0.04 | 0.08 |
Conditionally background territory | ||
Russula xerampelina | 0.03 | 0.06 |
Suillus variegatus | 0.07 | 0.13 |
Leccinum scabrum | 0.17 | 0.34 |
The total carcinogenic risk due to only two identified carcinogens for man – for the contaminated area is 0.1–2.1 × 10–2, and for conditionally background it is 0.3–3.4 × 10–2. According to the international criterion scale (Onishchenko et al., 2002) the risk is assessed as high unacceptable for production conditions, and requires taking remedial action or decrease.
Eating mycological products that have the following pollution levels: Cd – 8.4–16.2 MAC; Zn – 6.5–11.0 MPC; Cu – 2.5–5.2 MAC; Pb – 8.4–16.2 MAC; Ni and Co – 1.5 MACs throughout life can lead to the increase in carcinogenic and non-carcinogenic health risks. However, when assessing carcinogenic risk, it should be borne in mind that the stochastic nature of the carcinogenic process, a long latent period, the differences in age sensitivity to the effects of carcinogens do not allow accurately predict the timing of development of malignant neoplasms in the population.
CONCLUSION
Our findings revealed that in modern ecological conditions that have developed within the region under investigation, it is necessary to revise the background areas for monitoring the contamination of forest soils. The intensity of accumulation of macromycetes by pollutants and their ability to concentrate or deconcentrate are equally influenced by both the biological features of a particular species of macromycetes and the geochemical specificity of the biotope. It was found that for fungi, growing in pine-birch with the admixture of oak forest plantations, the contamination of fruit bodies with Zn, Cu and Cd is most likely. Mushroom raw materials harvested within both technologically contaminated and conditionally background areas are not safe, and its consumption as food can lead to the increase in carcinogenic and non-carcinogenic risks of the incidence of the population.
The research was carried out within the framework of the project “Support to regional and local development of adjacent territories of Belarus and Ukraine” using the equipment of the biochemistry laboratory of the State Scientific Establishment “The Polesie Agrarian Ecological Institute of the National Academy of Sciences of Belarus”.
Список литературы
Alonso J., Garcia M.A., Perez-Lopez M., Melgar M.J. The concentrations and bioconcentration factors of copper and zinc in edible mushrooms. Arch. Environ. Contam. Toxicol. 2003. V. 44. P. 180–188. https://doi.org/10.1007/s00244-002-2051-0
Baginsky V.F. Complex productivity of forest lands. Gomel, Institut lesa NAN Belarusi, 2007 (in Russ.).
Bakaytis V.I. Martene E.V., Belokrylova L.V., Basalaeva S.N. The content of heavy metals in edible mushrooms of the Novosibirsk Region and Altai Territory. Khranenie i pererabotka selhozproduktsii. 2006. V. 5. C. 38–41 (in Russ.).
Borovička J., Řanda Z. Distribution of iron, cobalt, zinc and selenium in macrofungi. Mycol. Progress. 2007. V. 6 (4). P. 249–259. https://doi.org/10.1007/s11557-007-0544-y
Brzezicha-Cirocka J., Mędyk M., Falandysz J., Szefer P. Bio- and toxic elements in edible wild mushrooms from two regions of potentially different environmental conditions in eastern Poland. Environ Sci. Pollut. Res. 2016. V. 23. P. 21517–21522. https://doi.org/10.1007/s11356-016-7371-0
Belyavskiy Yu.A., Nadtochij P.P., Myslyva T.M., Bigula S.M. Atlas of the distribution of heavy metals in the fruit bodies of macromycetes: pratice adviser. Zhytomyr, Evenok, 2016 (in Ukr.).
EFSA Journal Guidance on selected default values to be used by the EFSA Scientific Committee. Scientific Panels and Units in the absence of actual measured. Eur. Food Safety Authority J. 2012. V. 10. P. 2579.
Elekes C.C., Busuioc G. Influence of chemical composition of soil on metal accumulation in edible mushroom species of Russula genus. Rev. Roum. Chim. 2013. V. 58 (7–8). P. 629–637.
Falandysz J., Lipka K., Kawano M., Brzostowski A., Dadej M., Jędrusiak A., Puzyn T. Mercury content and its bioconcentration factors in wild mushrooms at Lukta and Morąg, Northeastern Poland. J. Agric. Food Chem. 2003. V. 51. P. 2830–2832. https://doi.org/10.1021/jf026016l
Georgescu A.A., Busuioc G. Determination of heavy metals in several species of wild mushrooms and their influence on peroxidase activity. Lucrări ştiinţifice. Seria Agronomie. 2011. V. 54 (2). P. 62–66.
Glazovskiy N.F. Biogeochemical circulation of substances in the biosphere. Moskva, Tovarishchestvo nauchnykh izdaniy KMK, 2006 (in Russ.).
GN 1.7.2041–06 The maximum permissible concentration (MPC) of chemicals in the soil. Postanovlenije Glavnogo gosudarstvennogo sanitarnogo vracha Rossijskoj Federatsii. 2006 (in Russ.).
GN 2.1.7.12–1–2004 List of maximum permissible concentrations (MPC) and approximate permissible concentrations (APC) of chemical substances in the soil. Postanovlenije Glavnogo gosudarstvennogo sanitarnogo vracha Respubliki Belarus. 2004 (in Russ.).
Ivanov A.I., Gorohova A.G., Andreeva M.I., Muhamedshyn R.K. Biological accumulation of cadmium by the fruit bodies of Agaricomycetes (Agaricomycetes). Mikologiya i fitopatologiya. 2017. V. 51 (3). P. 158–167 (in Russ.).
Ivanov A.I., Kostychev A.A., Skobanev O.V. Accumulation of heavy metals and arsenic by basidiomyomas of macromycetes of various trophic and taxonomic groups. Povolzhskiy ekologicheskiy zhurnal. 2008. V. 3. P. 190–199 (in Russ.).
Kakareka S.V., Kuharchik T.I. Sources of heavy metal emissions in Belarus. Minsk, Institut prirodopolzovanija NAN Belarusi, 2012. P. 1–28 (in Russ.).
Koroleva Yu.V., Ohrimenko M.A. Peculiarities of accumulation of heavy metals by forest mushrooms of the Kaliningrad region. Vestnik Baltiyskogo federalnogo universiteta imeni I. Kanta. 2015. V. 1. P. 106–117 (in Russ.).
Koshedub T.I. Wild macromycetes as a source of phosphorus in the diet of the population of Belarus. Problemy zdorovyya i ekologii. 2016. V. 2 (48). P. 86–89 (in Russ.).
Kula I., Solak M.H., Uğurlu M., Ișıloğlu M., Arslan Y. Determination of mercury, cadmium, lead, zinc, selenium and iron by ICP-OES in mushroom samples from around thermal power plant in Muğla, Turkey. Environ. Contam. Toxicol. 2011. V. 87 (3). P. 276–281. https://doi.org/10.1007/s00128-011-0357-1
Matveev A.V., Bordon V.E. Geochemistry of tertiary sediments of Belarus. Minsk, Navuka, 2013 (in Russ.).
Mikhalchuk N.V. Mobile forms of heavy metals and microelements in soils of carbonate series in the south-west of Belarus. Vestnik Natsionalnoy akademii nauk Belarusi. Ser. Khimicheskie nauki. 2017. V. 3. P. 90–97 (in Russ.).
Mikhalchuk N.V., Dashkevich M.M., Galuts O.A. The levels of heavy metals in the background and agrotechnogenic soils of the south-west of Belarus. Sbornik dokladov Mezhdunarodnoy nauchnoy konferentsii. Minsk, 2016. P. 268–271 (in Russ.).
Mleczek M., Magdziak Z., Goliński P., Siwulski M., Stuper-Szablewska K. Concentrations of minerals in selected edible mushroom species growing in Poland and their effect on human health. Acta Sci. Pol. Technol. Aliment. 2013. V. 12 (2). P. 203–214.
Mleczek M., Siwulski M., Stuper-Szablewska K., Rissmann I., Sobieralski K., Goliński P. Accumulation of elements by edible mushroom species: Part I. Problem of trace element toxicity in mushrooms. Journal of Environmental Science and Health, Part B: Pesticides, Food Contaminants, and Agricultural Wastes. 2013. V. 48 (1). P. 69–81. https://doi.org/10.1080/03601234.2012.716733
Murati E., Hristovski S., Melovski L., Karadelev M. Heavy metals content in Amanita pantherina in a vicinity of the thermo-electric power plant Oslomej, Republic of Macedonia. Fresenius Environmental Bulletin. 2015. V. 24 (5b). P. 1981–1984.
Myslyva T.M. Lead and cadmium in soils of agrolandscapes of Zhytomyr Polissya. Visnyk SNAU. V. 3 (25) P. 43–50 (in Ukr.).
Myslyva T.N., Belyavskiy Yu.A. An accumulation of heavy metals by wild edible macromycetes growing on the territory of Ukrainian Polessye and consumption health risk. Mikologiya i fitopatologiya. 2016. V. 50 (4). P. 241–249 (in Russ.).
Onishchenko G.G., Novikov S.M., Rahmanin Yu.A., Avaliani S.L., Bushtueva K.A. Bases of risk estimation for the health of population at the influence of chemical matters, contaminating an environment. Moskva, 2002 (in Russ.).
Pelgunov A.N., Pelgunova L.A. Heavy metal accumulation by fungi in the National Park “Lake Pleshcheyevo”. Povolzhskij ekologicheskij zhurnal. 2015. V. 2. P. 215–219 (in Russ.).
Pelkonen R., Alfthan G., Järvinen O. Cadmium, lead, arsenic and nickel in wild edible mushrooms. Helsinki, Finnish Environment Institute, 2006.
R 2.1.10.1920-04 Human health risk assessment from environmental chemicals. Moskva, Federalnyi tsentr gossanepidnadzora Minzdrava Rossii. 2004 (in Russ.).
Sazanova K.V., Velikova V.D., Stolyarova N.V. Accumulation of heavy metals by mushrooms. Ecological and species specificity, mechanisms of accumulation, risk for human health. Biomeditsinskiy zhurnal. 2017. V. 18. P. 336–361 (in Russ.).
Sesli E., Tuzen M., Soylak M. Evaluation of trace metal contents of some wild edible mushrooms from Black sea region, Turkey. J. Hazardous Materials. 2008. V. 160. P. 462–567. https://doi.org/10.1016/j.jhazmat.2008.03.020
Sibirkina A.P. Accumulation of manganese by pine-tree mushrooms in the conditions of Semipalatinsk Irtysh. Izvestija Omskogo GAU. 2009. V. 22. P. 299–301 (in Russ.).
Solomko T.F., Grodzinskaja A.A., Pashchenko L.A., Pchelintseva R.K. The mineral composition of some species of cultivated and wild mushrooms of the class Basidiomycetes. Mikologiya i fitopatologiya. 1986. V. 6. P. 474–478 (in Russ.).
Stankeviciene D. Heavy metals in agaricoid fungi from a deciduous forest under direct human impact. Budapest, Hungary. Metal ions in biology and medicine: proceedings of the 8th International symposium on metal ions in biology and medicine. 2004.
Svoboda L., Chrastny V. Contents of eight trace elements in edible mushrooms from a rural area. Food Additives and Contaminants. 2007. V. 25 (01). P. 51–58.
Szubstarska J., Jarzyńska G., Falandysz J. Trace elements in Variegated Bolete (Suillus variegatus) fungi. Chemical Papers – Slovak Academy of Sciences. 2012. V. 66 (11). P. 1026–1031. https://link.springer.com/article/10.2478/s11696-012-0216-5.
Tsvetnova O.B., Shatrova N.M., Shcheglov A.M. Accumulation of radionuclides and heavy metals by the fungal complex of forest ecosystems. Nauchnye trudy Instituta yadernykh issledovaniy. Kiev, 2001. P. 171–176 (in Russ.).
Wasser S.P., Beregovaya T.V., Bilaj V.T., Syrchin S.A. Macromycetes: medicinal properties and biological features. Kiev, 2012 (in Russ.).
World Abstract Soil Resource Base 2014. International soil classification system for soil diagnostics and creation of soil map legends. (WRB 2014). Rome, 2018 (in Russ.).
Yashchenko O.V. Food and biological role edible and medicinal mushrooms in the nutrition. Gigiena naselenyh mists. 2012. V. 59. P. 234–240 (in Ukr.).
Záhorcová Z., Árvay J., Hauptvogl M., Tomáš J., Haran L. Heavy metals determination in edible wild mushrooms growing in former mining area – Slovakia: health risk assessment. Potravinarstvo. 2016. V. 10 (1). P. 37–46.
Zhang D., Zhang Y., Morawska E., Bielawski L., Krasińska G., Drewnowska M., Pankavec S., Szymańska K., Falandysz J. Trace Elements in Leccinum scabrum мushrooms and topsoils from Kłodzka Dale in Sudety мountains, Poland. J. Mt. Sci. 2013. V. 10 (4). P. 621–627.
Zsigmond A.R., Varga K., Harangi S., Baranya E., Urák I. Elemental profile of edible mushrooms from a forest near a major Romanian city. Acta Universitatis Sapientiae: agriculture and environment. 2015. V. 7. P. 98–107. https://doi.org/10.1515/ausae-2015-0009
Багинский В.Ф. (Baginskiy) Комплексная продуктивность земель лесного фонда. Гомель: Институт леса НАН Беларуси, 2007. 295 с.
Бакайтис В.И., Мартене Е.В., Белокрылова Л.В., Басалаева С.Н. (Bakaytis et al.) Содержание тяжелых металлов в съедобных грибах Новосибирской области и Алтайского края // Хранение и переработка сельхозпродукции. 2006. № 5. С. 38–41.
Білявський Ю.А., Надточій П.П., Мислива Т.М., Бігула С.М. (Belyavsky et al.) Атлас розподілу важких металів у плодових тілах макроміцетів: практ. порадник. Житомир: Вид-во О. О. Євенок, 2016. 48 с.
Вассер С.П., Береговая Т.В., Билай В.Т., Сырчин С.А. (Wasser et al.) Макромицеты: лекарственные свойства и биологические особенности. К.: Наш формат, 2012. 285 с.
Глазовский Н.Ф. (Glazovskiy) Биогеохимический круговорот веществ в биосфере. М.: Товарищество научных изданий КМК, 2006. С. 56–64.
Иванов А.И., Горохова А.Г., Андреева М.И., Мухамедшин Р.К. (Ivanov et al.) Биологическая аккумуляция кадмия плодовыми телами агарикомицетов (Agaricomycetes) // Микология и фитопатология. 2017. Т. 51. Вып. 3. С. 158–167.
Иванов А.И., Костычев А.А., Скобанев О.В. (Ivanov et al.) Аккумуляция тяжелых металлов и мышьяка базидиомами макромицетов различных трофических и таксономических групп // Поволжск. экол. журн. 2008. № 3. С. 190–199.
Какарека С.В., Кухарчик Т.И. (Kakareka, Kukharchik) Источники выбросов тяжелых металлов в Беларуси. Минск: Ин-т природопользования НАН Беларуси, 2012. С. 1–28.
Кожедуб Т.И. (Kozhedub) Дикорастущие макромицеты как источник фосфора в питании населения Беларуси // Проблемы здоровья и экологии. 2016. №2(48). С. 86–89.
Королева Ю.В., Охрименко М.А. (Koroleva, Okhrimenko) Особенности накопления тяжелых металлов лесными грибами Калининградской области // Вестник Балтийского федерального университета им. И. Канта. 2015. Вып. 1. С. 106–117.
Матвеев А.В., Бордон В.Е. (Matveev, Bordon) Геохимия четвертичных отложений Беларуси. Минск: Беларус. навука, 2013. 191 с.
Мировая реферативная база почвенных ресурсов 2014. Международная система почвенной классификации для диагностики почв и создания легенд почвенных карт (WRB 2014). Рим, 2018. 216 с.
Мислива Т.М. (Myslyva) Свинець і кадмій у ґрунтах агроландшафтів Житомирського Полісся // Вісник Сумського національного аграрного університету. 2013. Вип. 3 (25). С. 43–50.
Михальчук Н.В. (Mikhalchuk) Подвижные формы тяжелых металлов и микроэлементов в почвах карбонатного ряда юго-запада Беларуси // Вестн. Нац. акад. навук Беларусі. Сер. хім. навук. 2017. № 3. С. 90–97.
Михальчук Н.В., Дашкевич М.М., Галуц О.А. (Mikhalchuk et al.) Уровни содержания тяжелых металлов в фоновых и агротехногенных почвах юго-запада Беларуси / Сборник докладов Международной научной конференции. Минск, 2016. Т. 1. С. 268–271.
Мыслыва Т.Н., Белявский Ю.А. (Myslyva, Belyavskiy) Накопление тяжелых металлов съедобными грибами Украинского Полесья и риски при употреблении их в пищу // Микология и фитопатология. 2016. Т. 50. Вып. 4. С. 241–249.
Онищенко Г.Г., Новиков С.М., Рахманин Ю.А., Авалиани С.Л., Буштуева К.А. (Onishchenko et al.) Основы оценки риска для здоровья населения при воздействии химических веществ, загрязняющих окружающую среду. М.: НИИ ЭЧ и ГОС, 2002. 408 с.
Пельгунов А.Н., Пельгунова Л.А. (Pelgunov, Pelgunova) Аккумуляция тяжелых металлов грибами на территории национального парка “Плещеево озеро” // Поволжский экологический журнал. 2015. № 2. С. 215–219.
Перечень предельно допустимых концентраций (ПДК) и ориентировочно допустимых концентраций (ОДК) химических веществ в почве. ГН 2.1.7.12-1-2004 (GN 2.1.7.12-1-2004) Постановление Главного государственного санитарного врача Республики Беларусь № 28 от 25.02.2004 г.
Предельно допустимые концентрации (ПДК) химических веществ в почве. ГН 2.1.7.2041–06 (GN 1.7.2041-06) Постановление Главного государственного санитарного врача Российской Федерации № 1 от 23.01. 2006 г.
Руководство по оценке риска для здоровья населения при воздействии химических веществ, загрязняющих окружающую среду Р 2.1.10.1920-04 (R 2.1.10.1920-04). М.: Федеральный центр госсанэпиднадзора Минздрава России, 2004. 143 с.
Сазанова К.В., Великова В.Д., Столярова Н.В. (Sazanova et al.) Накопление тяжелых металлов грибами. Экологическая и видовая специфичность, механизмы аккумуляции, потенциальная опасность для человека // Биомедицинский журнал. 2017. Т. 18. С. 336–361.
Сибиркина А.Р. (Sibirkina) Аккумуляция марганца грибами соснового бора в условиях Семипалатинского Прииртышья // Известия ОГАУ. 2009. № 22. С. 299–301.
Соломко Э.Ф., Гродзинская А.А., Пащенко Л.А., Пчелинцева Р.К. (Solomko et al.) Минеральный состав некоторых видов культивируемых и дикорастущих грибов класса Basidiomycetes // Микология и фитопатология. 1986. № 6. С. 474–478.
Цветнова О.Б., Шатрова Н.М., Щеглов A.M. (Tsvetnova et al.) Накопление радионуклидов и тяжелых металлов грибным комплексом лесных экосистем // Науч. тр. Ин-та ядерных исследований. Киев, 2001. № 3. С. 171–176.
Ященко О.В. (Yashchenko) Харчова та біологічна роль їстівних і лікарських грибів в харчуванні населення // Гігієна населених місць. 2012. № 59. С. 234–240.
Дополнительные материалы отсутствуют.
Инструменты
Микология и фитопатология