Ботанический журнал, 2022, T. 107, № 4, стр. 385-396
PHOTOSENSITIVITY OF SEED GERMINATION OF CYMBIDIUM DAYANUM (ORCHIDACEAE)
A. V. Balashova 1, E. V. Andronova 1, *, A. A. Kovaleva 1, O. G. Butuzova 1
1 Komarov Botanical Institute of RAS
197376 St. Petersburg, Prof. Popova Str., 2, Russia
* E-mail: elena.andronova@mail.ru
Поступила в редакцию 26.02.2021
После доработки 12.01.2022
Принята к публикации 25.01.2022
- EDN: EUXSLJ
- DOI: 10.31857/S0006813622040020
Аннотация
The study of the seed germination and the development in vitro of rare white-flowered form of Cymbidium dayanum was carried out. The seeds were germinated in conditions of darkness and light, at 20°C and with a transfer to 26°C in the 10th week of cultivation. Seed germination was successful at both 20°C and 26°C, however optimal temperature for development of the seedlings was 26°C. At 20°C in the light, seed germination began in the 20th week after sowing, whereas in the darkness in the 5th week. Thus, the light significantly increased the time before germination. In addition, the germination rate slowed down five weeks after the cultures transfer to 26°C and to the light, and by the end of the experiment, the percentage of germination was 80% compared with 94% in the dark. The results indicate a negative effect of light on the duration of germination suggesting its photosensitivity. The unusual type of germination was identified. The protocorm emergence took place from the chalazal end of the seed, instead of its middle part as in most orchids.
Many tropical orchid species have been introduced and successfully propagated in culture, both vegetatively and by means of seeds, using the method of germination on a nutrient medium in culture in vitro. Some orchids are known to be characterized by difficult seed germination, the mechanism of which is not always clear (see review by Andronova et al., 2009). Among the factors influencing the success of an orchid seed germination, the most important ones are the composition of nutrient media, temperature regime and light (Arditti, 1967; Fast, 1982; Rasmussen, 1995; Cherevchenko et al., 2008). The point of view on the taxon-specificity of seed germination conditions is currently supported. The factors affecting germination are likely differed in various species, subspecies, forms, and varieties, as it was demonstrated for other groups of flowering plants (Nikolaeva et al., 1999).
Cymbidium dayanum Rchb. f. is a tropical orchid species native to Southeast Asia. It is a highly decorative species that is in demand by orchid lovers and is very popular in the flower market in Asia (De, 2020; Nahar et al., 2013). The quantity of C. dayanum in nature is decreasing due to negative anthropogenic impact (Kumar et al., 2018). Since 1990 it has been listed in the Red Book of India (Nongdam, Chongtham, 2012).
Plants of C. dayanum are epiphytic or lithophytic, growing on trees and on steep rocky slopes (Pridgeon et al., 1999; Pratt, Bio, 2012; Averyanov et al., 2018). Usually, the flowers have white tepals with a longitudinal purple stripe and a purple with a yellow stripe three-lobed labium (Hartini, Wawangningrum, 2019; Matsuda, Sugiura, 2019). The form with pure white labium and tepals is very rare.
There is information on the germination of seeds and the cultivation of seedlings of some Cymbidium species including C. dayanum in sterile in vitro culture (Matsui et al., 1970; Prasad, Mitra, 1975; Paek, Murthy, 2002; Chang et al., 2005; Cherevchenko et al., 2008; Yuanhua et al., 2008; Nongdam, Chongtham, 2012; Mahendran et al., 2013). However, no special studies on the effect of temperature and light on the germination of C. dayanum seeds were carried out. The data on seed germination and in vitro cultivation of the rare white-flowered form of C. dayanum is completely absent in the literature.
The aim of the present study was to investigate the influence of cultivation conditions on the seed germination and the peculiarities of plant development of plants in vitro of the white-flowered form of C. dayanum.
MATERIALS AND METHODS
The seeds of C. dayanum from a single seed capsule were used for planting. It was cut on 16.10.2017 from a plant in a private collection. The date of pollination and the type of crossing (self-pollination or cross-pollination) are unknown. The term of fruit development was presumably 1 year. The seeds were sown on 21.11.2017.
A modified nutrient medium according to Harvais (1973) was used for germination of the seeds: Ca(NO3)2 · 4H2O – 400 mg/l, KH2PO4 – 200 mg/l, KCl – 100 mg/l, KNO3 – 200 mg/l, MgSO4 · 7H2O – 200 mg/l, NH4NO3 – 400 mg/l, FeSO4 · 7H2O – 27.8 mg/l, Na2EDTA – 37.3 mg/l, MnSO4 4H2O – 0.5 mg/l, H3BO3 – 0.5 mg/l, ZnSO4 · 7H2O – 0.5 mg/l, CuSO4 · 5H2O – 0.5 mg/l; vitamins: nicotinamide (PP) – 1 mg/l, thiamine (B1) – 1 mg/l; pyridoxine (B6) – 1 mg/l; grated potatoes – 90 g/l, sucrose – 20 g/l, agar-agar – 8 g/l.
Before sowing, the fruit was thoroughly washed with soap and rinsed under running water, then it was immersed in 96% ethanol and burned in the flame of an alcohol burner. After that, it was placed for 30 minutes in a disinfectant solution consisting of 100 ml of bleach and 150 ml of distilled water. Then it was washed with sterile distilled water three times for 10 minutes each.
Sterile plastic Petri dishes (90 ml) were used. The seeds were removed from the capsule and sown on the surface of the nutrient medium. In total, 10 dishes were used with 200–300 seeds in each. Eight dishes were placed in the dark, and two were exposed to constant light at 1000 lx (fluorescent lighting of 2850 lm). Germination of seeds both in the dark and in the light was carried out at temperature of 20°C. The seed germination experiment lasted 26 weeks in total, but some variants were completed in the 20th week.
For some part of the seedlings kept in the darkness at 20°C from the beginning, the growing conditions were changed after 10 weeks of cultivation. They were transferred to 26°C. At the same time, some of them were placed in conditions of darkness, while others were placed under light (photoperiod 16/8 hours day/night). Two Petri dishes were used in each of them. The cultures were observed once a week.
The structure of seeds at the time of sowing on a nutrient medium, as well as that of germinating seeds and protocorms at the early stages of their development, was studied in the 10th week (one dish with 200–300 seeds from darkness at 20°C) using the method of clearing in methyl benzoate. For this purpose, the material was fixed in 70% ethanol or in a FAA mixture consisting of 70% ethanol, 45% formalin, and glacial acetic acid in a ratio of 100: 7: 7, respectively. Then it was washed from the fixative and dehydrated through a series of alcohols with increasing concentration. Then it was passed through a series of three mixtures of 96% ethanol and methyl benzoate (in a ratio of 2: 1; 1: 1; 1: 2, respectively). The material was kept in each liquid for 10 minutes, at the end it was poured with pure methyl benzoate. Then the material was transferred to a glass slide in a drop of methyl benzoate and covered with a cover glass, which was sealed at the edges with colorless nail polish. Immediately after that, the slides were examined and photographed using an Axioplan-2 MOT microscope (Zeiss). An AxioCam MRc 5 camera (Zeiss) and the AxioVision 4.8 software (Zeiss) were used to take images.
Size measurements of 30–100 embryos and protocorms were carried out every week for 20 weeks from the moment of sowing the seeds. The length and width were measured on unfixed material, without opening the Petri dish, using a Stemy 2010 Zeiss stereoscopic microscope and the Image-Pro Insight 8.0 software package.
Analysis of variance and pairwise comparison of data samples were used for statistical processing of the results. Pairwise comparison of morphometric parameters was performed based on the parametric Student’s t-criterion; for multiple comparisons, univariate dispersive analysis was used, taking into account the Bonferroni correction. Differences in the percentage of germination in different variants of the experiment were calculated similarly, but for pairwise comparisons of specimens, the nonparametric Mann-Whitney U test was used.
To assess the influence of environmental factors (temperature and light) on sizes and on the percentage of seed germination, two-factor analysis was used. Statistical analysis was carried out using the Microsoft Office Excel 2003, PAST and RStudio software package. The statistical significance of the differences was established at a probability level of 0.5.
RESULTS
Seed morphology
The seeds had an oblong spindle-shaped shape (Fig. 1, 1). The embryo was very small with a branched suspensor. The volume of the embryo was much smaller than the volume inside the seed cavity where it was located (Fig. 1, 1).
Fig. 1.
Seeds of Cymbidium dayanum after sowing (1, clearing in methyl benzoate) and during germination (2–8, unfixed materials): 2 – 1 week after sowing, 3, 4 – after 5 weeks in the darkness (3) and in the light (4), 5, 6 – after 10 weeks in the darkness (5) and in the light (6), 7, 8 – after 20 weeks in the darkness (7) and in the light (8). Scale bars: 1 – 200 μm, 2–8 – 1 mm.
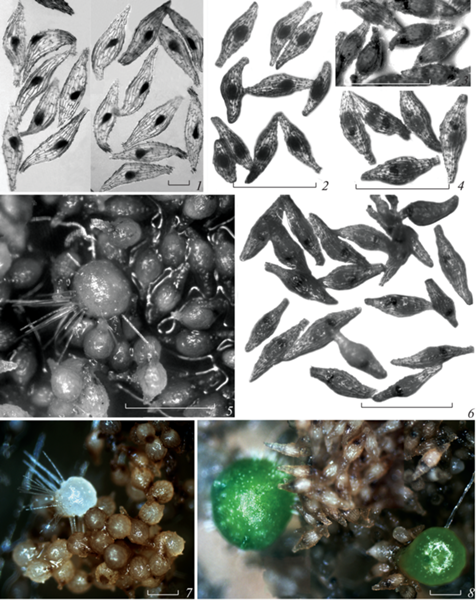
The embryos were predominantly oviform (Fig. 1, 1, 2, 1), however, the seeds with embryos of spherical and ellipsoidal shapes were sometimes found (Fig. 1, 1).
The seed length was 818.9 ± 60.7 μm in average, the embryo length and width were 130.8 ± 17.6 μm and 72.3 ± 10.9 μm.
The seeds used in this study were mature and completely formed.
Seed morphology during germination
The seeds began to swell after sowing. During the first week of cultivation, the embryo size increased slightly under all conditions (Fig. 1, 2), the embryo’s suspensor turned black and was clearly visible under a microscope (Fig. 2). Within a few weeks, the embryo was growing inside the seed and no rupture of the seed coat was noted (Fig. 2, 2–10). The sizes of the embryo increased gradually, due to both the cell expansion in its basal and central parts, and cell division in its apical part. The embryo width growth was insufficient to rupture the seed coat in central part of the seed. Germination was occurred through the chalazal end as a result of the embryo growth along the apical-basal axis (Fig. 2, 11, 12).
Fig. 2.
Change of seed morphology in Cymbidium dayanum during germination (clearing in methyl benzoate). 1 – seed at the moment of sowing on a nutrient medium, 2–10 – intraseminal growth of the embryo, 11–13 – protocorm emergence from the seed coat. Scale bars: 100 μm. rd – rhizoid, s – suspensor.
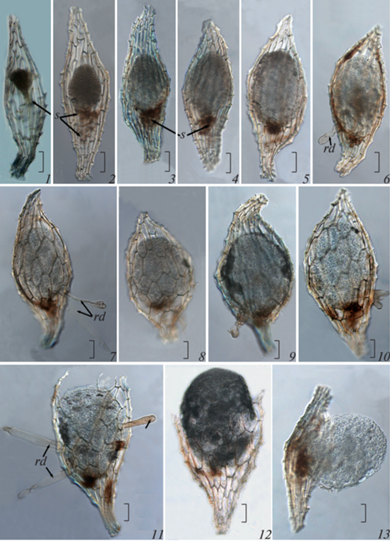
At the moment of emergence from the seed coat, the protocorm was ovoid (Fig. 2, 11). At that stage of development, histological differentiation of cells was observed in it. In the apical part, the cells were comparatively smaller, closely adjacent to each other, and comparatively larger in the basal part.
The formation of hairs occurred at different stages of germination depending on experimental conditions. Under darkness, the first hairs could often be found in the embryo growing inside the seed, even before its complete release from the seed coat (Fig. 2, 6, 7, 9, 10). Under light, they were formed after germination at the protocorm stage. At the early developmental stages, the hairs were usually uniseriate (Fig. 2, 6, 11). With the development of the protocorm, the number of epidermal hairs increased, and they were multiseriate (Fig. 1, 5, 7, 8).
Germinated orchid seeds are hardly distinguished from non-germinated ones. We considered the seed germinated if there was a rapture in the seed coat or the appearance of epidermal hairs was observed. The first signs of seed germination were noted in the 5th week in the dark (Fig. 1, 3), and only in the 15th week after sowing in light (Fig. 1, 4, 6).
At the moment of emergence from the seed coat, the protocorm shape was ovoid regardless of the cultivation conditions. Subsequently, the protocorms which developed in the light acquired an oblong or pear-like shape. After the leaf primordium formation, the protocorm shape became spherical or ovoid under all experimental conditions.
Hairs in the protocorms that developed in the light generally appeared later and in smaller numbers than in the protocorms that grew in the dark. They were relatively short (not longer than the radius of the protocorm itself, but in most cases even shorter). In the protocorms that developed in the dark, the length of the hairs often exceeded their diameter.
Changing the percentage of seed germination
Data on the dynamics of seed germination depending on the cultivation conditions are shown in Fig. 3.
Fig. 3.
Dynamics of germination of the Cymbidium dayanum seeds. The Y-axis shows the percentage of seed germination, the X‑axis shows the weeks after sowing the seeds. At p < 0.05, statistically significant differences between variants A and B appeared from the 5th to the 26th week; between A and C from the 12th to the 14th week; between A and D from 20th to the 26th week; between C and D from the 12th to the 14th week, and in the 20th week.
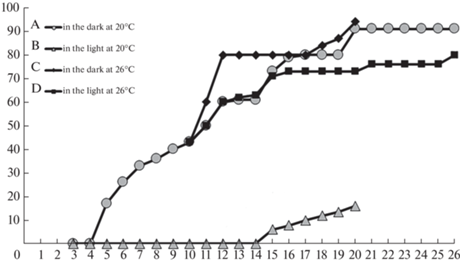
The maximum percentage of seed germination was marked only under darkness. In the 20th week of cultivation at 26°C, it was 94%, and at 20°C – 91%. Despite some difference between the values noted above, there were no significant differences based on the Student’s t-criterion between them.
After the cultures were transferred from dark conditions to light and from 20 to 26°C, the percentage of germination in the 26th week was only 80%, this was significantly lower than in the variants in the darkness both at 20 and 26°C.
The lowest percentage of seed germination was observed in the variant under light at 20°C. In the 20th week, it was only 16%. The differences between it and the percentage of seed germination in all other variants of the experiment occurred to be statistically significant.
Morphometric analysis during seed germination
The length and width of the embryos changed in different ways under dark and light. However, the most informative for comparison were the data from the 1st to the 10th week of cultivation, since during this period the most important processes, such as the swelling and intraseminal growth of the embryo, as well as the release of protocorms from the seed coat took place. In this regard, the sizes of embryos and protocorms are presented in diagrams up to the 10th week of cultivation (Fig. 4), and these values in the following weeks are given in the text.
Fig. 4.
Changes in the size of embryos and protocorms during germination of Cymbidium dayanum seeds at 20°C under darkness (a) and light (b). The Y-axis shows the length and width (mm), the X-axis – the weeks after sowing the seeds. * – differences between sizes in the light and in the dark are statistically significant at p < 0.05.
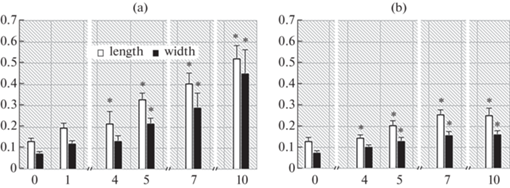
Sizes of embryos cultured in the darkness change significantly (Mann-Whitney test) after first week. In this case, the mean value of the embryo length changed from 0.130 mm to 0.195 mm, the mean value of its width from 0.072 mm to 0.120 mm. During the 2nd, 3rd and 4th weeks after sowing, they also increased, but not considerably. Thus, in the 4th week of the experiment in the darkness, the average embryo length was 0.21 mm and its width was 0.13 mm.
The sizes of the embryos exposed to the light did not practically change in the first 4 weeks of cultivation. By the 4th week, the length increased from 0.130 mm to 0.140 mm, and the width increased from 0.072 mm to 0.100 mm.
Thus, in the first weeks after sowing, a more intensive growth of the embryos was observed under the dark than under the light. Differences in the parameters of embryos germinating in the light and in the darkness were reliable at p < 0.05.
From the 4th to the 10th weeks of cultivation in the darkness, the length and width of embryos and protocorms gradually increased. By the 10th week, when 50% of the seeds were already germinated (Fig. 1, 5), the length was 0.52 mm, and the width was 0.45 mm.
From the 4th to the 10th weeks of cultivation at the light, the length and width of the embryos and protocorms increased insignificantly. By the 10th week, the length was 0.25 mm and the width 0.16 mm. These parameters under light were 2–3 times less than those in the darkness (Fig. 1, 5, 6).
The differences in the sizes of embryos and protocorms in the dark and in the light, beginning from the 1st week of the experiment were statistically significant. The most considerable differences in length and width were observed from the 5th to the 10th week.
From the 10th to the 12th week of cultivation in the darkness, the measured parameters did not practically change, and from the 12 th to 16 th week there was a distinct protocorm growth, as a result the average value of length and width increased to 1.87 and 1.75 mm, respectively. From the 16th to 18th week of cultivation, the average value of the length and width of the protocorms practically did not change.
In the variant of the experiment at 20°C, the average sizes of the protocorms were higher when the experiment was carried out in the darkness. By the 18th week of the experiment, the average length and width of the protocorms under the light exposition was 1.4 and 1.3 mm, and in the darkness 1.9 and 1.8 mm, respectively.
With an increase in the cultivation time, the heterogeneity of the sizes of the measured structures increased, which was expressed in an increase in the index of standard deviation from the average. Its value was especially high in the variant of the experiment in the darkness. This is due to the increase in heterogeneity of the sample in the 13th–18th weeks of cultivation under dark, since it included both non-germinated seeds and protocorms at different stages of development (some of which were formed 9 weeks ago). In the variant of seed germination in the light, only single protocorms were formed, and even in the 20th week after sowing, most of the seeds remained non-germinated (Fig. 1, 8).
Influence of light on seed germination after transferring from 20°C to 26°C
A part of the cultures, which were kept for 10 weeks in the darkness at 20°C, was transferred to the climatic chamber where the cultivation temperature was increased to 26°C. Some of the cultures were placed in the dark, and their other part in the light (photoperiod 16/8 hours day/night). At the date of this experiment beginning, the seeds were swollen or germinated, the percentage of seed germination was about 50%. In the 20th week at 26°C, it reached 70% in the light, and 94% in the darkness. In the 26th week in the light, the percentage of seed germination was 80%, in the darkness it remained 94% (Fig. 3).
An increase of the cultivation temperature, while maintaining dark conditions, led to a drastic increase in the percentage of seed germination already in the 1st week after changing the conditions, i.e. led to acceleration of the germination rate. After 2 weeks under the new conditions, the percentage of germination remained practically unchanged or changed more smoothly. The maximum percentage was reached in the dark 10 weeks after the rising of the cultivation temperature, i.e. in the 20th week after sowing seeds, as in the variant at 20°C. Herewith the maximum percentage of germinated seeds was approximately the same at both 26 and 20°C.
Figure 3 demonstrates that after transfer to a temperature 26°C, the percentage of germination was lower in light conditions compared to dark conditions for all weeks of its measurement.
The graphs of the change in the percentage of seed germination at 26°C in the light and at 20°C in the dark for 5 weeks coincided, i.e. germination rates remained similar after changing the cultivation conditions. Subsequently, the rate of germination in the light decreased markedly.
The highest percentage of germination was observed at all stages of cultivation in the dark as compared to light conditions. Obviously, the reason for the differences in results in the dark and in the light is not related to the degree of seed viability but is determined by the inhibitory effect of light on the rate of germination.
From the 10th to the 12th week, the sizes of embryos and protocorms practically did not change after transfer from darkness and 20°C to new conditions. Apparently, a slight change in the size of the protocorms was not the result of stress after an increase in the cultivation temperature, since during the same period the growth of seedlings left under the same conditions was also insignificant.
After the 12th week, the growth of protocorms cultured at 26°C, both in the light and in the darkness, increased dramatically. By the 15th week, the average length of protocorms kept in the darkness increased from 0.45 mm to 2.25 mm, and the width increased from 0.3 mm to 1.6 mm. Up to the 18th week, the values of these parameters also increased, but more smoothly, reaching the following values: length 3.2 mm and width 3 mm. The mean of the sizes of the protocorms exposed to light were lower than in the darkness (Fig. 5), however, the differences were statistically insignificant.
Fig. 5.
Changes in the size of embryos and protocorms during germination of Cymbidium dayanum seeds 26°C in the dark (a) and in the light (b). During the first 10 weeks, the experiment was conducted in the dark and at 20°C. The Y-axis shows the length and width in mm, the X-axis shows the weeks after sowing the seeds.
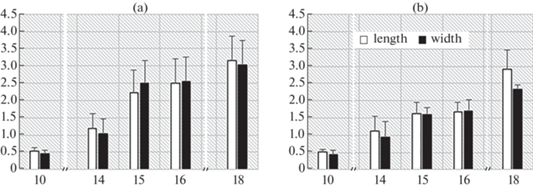
In general, by the end of 18 weeks after sowing, the percentage of germination was 70–85%, the length and width of the protocorms growing at 26°C was significantly higher than of those at 20°C. Moreover, significant differences were established precisely between the sizes of protocorms cultivated at different temperatures, and they did not depend on the presence or absence of light. The maximum length of protocorms was observed in the darkness at a temperature of 26°C (3.16 ± 0.8 mm), the minimum – under constant artificial light and 20°C (1.42 ± 0.3 mm). The results obtained indicate a positive effect of a temperature of 26°C on the dynamics of an increase in the sizes of protocorms during cultivation.
When the seeds were germinated in both darkness and light, the embryos were white. In this regard, C. dayanum can be distinguished as leuсoembryophyte, according to the concept of M.S. Yakovlev and G.Ya. Zhukova (1973). The seedlings forming in darkness and their leaves also were white (Fig. 6, 1, 1a); they obtained a green color only after transfer to the light (Fig. 6, 2).
Fig. 6.
Influence of light on seed germination after transferring from 20°C to 26°C. 1–6 – cultures after 26 weeks of experiment at the darkness (1, 1a) and at the light (2–6). Some fragments of photo 2 are given on a larger scale (3–6). The arrows mark the places of initiation of the primordium of the first leaf. It can be seen that the apical part of the protocorm turns green at the stage of initiation of the primary leaf. Scale bars: 1 mm.
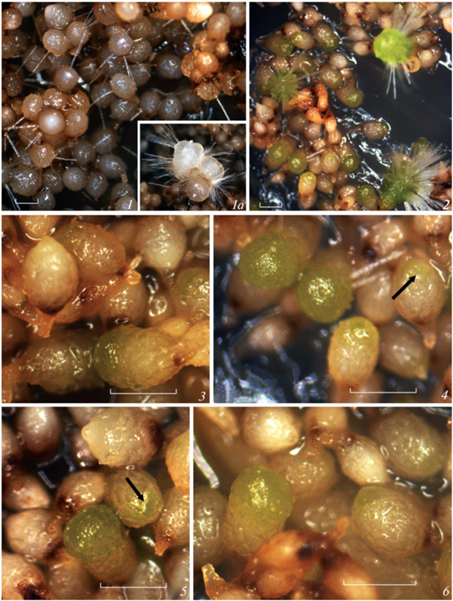
When the seeds germinated under the light, the chlorophyll appeared in the apical part of the protocorm at the stage of the first leaf primordium initiation (Fig. 6, 2–6). The rest part of the protocorm below the first shoot node never turned green. Thereby, the chlorophyll appeared already in the post-seed period and only at a certain stage of seedling development. This distinguishes the studied orchid species from the other epiphytic orchids in which the embryo in the process of germination under the light and undifferentiated protocorms are already chlorophyllous, for example those in Epidendrum fulgens (Voges et al., 2014).
During subsequent development, in some protocorms both in the dark and in the light, the formation of 2–3 shoot apices with leaf primordia or several protocorm-like structures was observed. However, in most cases, only one protocorm with a single shoot apex developed from the embryo.
DISCUSSION
According to the literature data, the length of a mature seed of C. dayanum ranges from 650 up to 900 μm, and the width from 200 to 300 μm (Chang et al., 2005). The embryo has spherical shape, its length and width are similar, about 130 ± 9 μm (Kolomeitseva et al., 2012). In our experiment, the embryo length did not practically differ from previously published, and the width of the embryo was 2 times less than its length, so the embryo was predominantly ovoid (Fig. 1, 1, 2, 1), however, seeds with embryos of spherical and ellipsoidal shapes were rarely found (Fig. 1, 1). Literature and our data indicate that C. dayanum shows a high degree of interspecific variability in the shape and sizes of an embryo.
Orchid seeds germinate not in the same way as the other angiosperms. According to M.G. Nikolaeva et al. (1999) “germination is considered as the pecking out the root from the seed coat”, usually in the hilum area. In orchids, the embryo has no embryonic root. As a rule, at the early stages of germination the orchid embryo evenly increases in size exclusively through cell growth, due to this, it ruptures the seed coat in the middle part of the seed (Rasmussen, 1995; Vinogradova, Andronova, 2002; Chang et al., 2005). The main role in this process is played by embryo growth in width only due to the cell expansion, but without their division. The seed coat ruptures when the embryo width exceeds the seed width. The embryo of C. dayanum in the present study was 2 times narrower than usually indicated for this species, however, it was located in the seeds that did not differ in size from the previously reported. In this regard, the growth of cells during the period of swelling, apparently, could not ensure the rupture of the seed coat, since the size of the swollen embryo did not exceed the width of the seed, and it remained inside it. After the embryo has swollen, the process of cell division begins. In the case of orchid embryo, the ability to division is characteristic only for some cells in its apical part. Despite opposing opinions on the presence or absence of the shoot apex in the embryo of orchids, it is widely accepted that the embryo has polarity by the apical-basal axis, and the location of the shoot apex can always be accurately indicated (Veyret, 1965, 1974). The shape of the embryo, its extremely small sizes especially compared to the volume of seed cavity, in which it is located, can affect the germination process. A small, narrow embryo, as in the present study, can germinate only due to its growth in length. In this case, the rupture of the seed coat and the protocorm emergence took place from the chalazal end of the seed, instead of its middle part. In the present study, an unusual type of germination was identified. We have not found other examples among orchids for which this type of germination was indicated. However, germination through the chalazal end of the seed was noted for some plants with underdeveloped embryos in mature seeds, for example in Fritillaria pallidiflora (Pozdova et al., 2008).
The duration of seed germination in C. dayanum slightly varied between different studies. According to Nongdam and Chongtham (2012), C. dayanum seeds began to germinate 2 weeks after sowing. In that study, immature seeds from capsules in the 5th month after pollination were used. According to Chang et al. (2005) the seeds of C. dayanum germinated after one month. These were mature seeds, from the capsules in the 12th month after pollination, which were sown on a nutrient medium immediately after cutting. In both studies, seed germination was carried out at 25°C and in the light. There was no variant in darkness in the experiments above mentioned. In our experiment, mature seeds were used, as in Chang et al. (2005), but from the fruit one and a half months after it was cut. Despite the fact that the germination temperature was 20°C, i.e. lower than those of the aforementioned researchers, the seeds also began to germinate one month after sowing. However, this was observed only under darkness. Surprisingly it was the lack of germination in the light up to 14 weeks of cultivation.
The composition of the nutrient medium is one of the factors affecting the success of germination of orchid seeds. Tropical orchid species are believed to be less difficult to cultivate in vitro than northern ones. For the latter, a suboptimal composition of the nutrient medium can be the reason for the absence of seed germination (Fast, 1982). For seed germination of the most tropical orchid species there are no specific requirements for the mineral composition of culture media (Arditti, 1982). In this regard, it is not surprising that media of different compositions were used for C. dayanum (Chang et al., 2005; Yuanhua et al., 2008; Nongdam, Chongtham, 2012). However, the percentage of seed germination on nutrient media of similar composition varied significantly. For example, in Nongdam and Chongtham (2012) the percentage of C. dayanum seed germination on MS medium was only 30%, while in Yuanhua et al. (2008) it was 98%. Probably, this may depend on the quality and state of the seeds, as well as on the cultivation conditions.
In the present study, a modified Harvais medium was used. It was developed for hardy Cypripedium (Harveis, 1973), but it turned out that it could be used for seed germination and cultivation of seedlings of Cymbidium dayanum as well, since the percentage of seed germination and seedling viability was very high.
The effect of temperature on the germination of orchid seeds has not been studied in detail. For many orchid species, temperature occurs to be the main seasonal signal regulating seed dormancy. The response to temperature is highly species-specific (Arditti, 1967; Rasmussen et al., 2015). For example, stressful exposure to high temperatures causes delayed germination in some species. With prolonged exposure to high temperatures (20–25°C), seeds can undergo secondary dormancy, or, conversely, the heat can promote germination (Johnson, Kane, 2012). Also, temperature has a significant effect on the rate of seed germination (Rasmussen, 1995).
Germination of orchids is possible in a rather wide temperature range, but maximum germination is achieved within narrow limits. For most species, the optimum temperature is considered to be 20–25°C (Arditti, 1982; Rasmussen, 1995; Kauth et al., 2008). For the members of Cymbidium genus, including C. dayanum, the seeds are usually germinated at 25°C (Paek, Murthy, 2002; Chang et al., 2005; Tawaro et al., 2008; Yuanhua et al., 2008; Nongdam, Chongtham, 2012; Mahendran et al., 2013). In our experiment, the maximum percentages of seed germination in C. dayanum practically did not differ under conditions of 20 and 26°C, and amounted to 91% and 94%, respectively. Thus, we can say that in the darkness, at both 20 and 26°C, seed germination proceeded successfully. This is not surprising since the temperatures used are in the 20–26°C range recommended for germination of tropical orchid species (Arditti, 1982). However, in the present study, the seeds germinated at different times, and the maximum percentage of germination was achieved in the dark in the 20th week after the start of the experiment. The maximum percentage of germination (98%) in the work of Yuanhua et al. (2008) was recorded in the 10th week after sowing the seeds and germinating in the light. The seeds we studied germinated for a longer time compared with the seeds of the same genus used in the experiments of foreign colleagues.
Analysis of dynamics of the protocorm sizes indicates that temperature is an important factor that affects their growth and development. The protocorms transferred to 26°C reached the maximum size. Obviously, this temperature, in comparison with 20°C, is optimal for growing seedlings of the white-flowered form of C. dayanum.
Multivariate dispersive analysis confirmed the effect of temperature only, but not light, on the change in the protocorms sizes during in vitro cultivation. No interaction of these 2 factors was noted.
Light is a key environmental factor that determines seed germination in some species, stimulating or inhibiting this process (Rasmussen, 1995). The phenomenon of photosensitivity is observed in seeds of many taxonomic groups (Nikolaeva et al., 1999); for orchids this problem remains poorly investigated. There are several points of view regarding the effect of light on the germination of orchid seeds. It is generally accepted that the seeds of epiphytic orchids germinate better in the light, and those of terrestrial ones in the darkness (Rasmussen et al., 2015). However, according to P.J. Kauth et al. (2008), this reaction is rather species-specific and does not depend on ecological characteristics of the species.
Experimental data on the effect of light on seed germination in the members of the genus Cymbidium are contradictory. In most cases, it is believed that Cymbidium seeds germinate better under conditions of a 16-hour photoperiod, however, there is also an opposite data about better germination in the darkness (Arditti, 1967; Paek, Murthy, 2002). For C. dayanum, studies on the effect of light on seed germination have not yet been carried out, and all known experiments were realized exclusively at the light (Chang et al., 2005; Yuanhua et al., 2008; Nongdam, Chongtham, 2012).
Analysis of the dynamics of seed germination of C. dayanum in the darkness and in the light indicates the inhibitory effect of light on seed germination. As follows from Figure 3, the seed germination percentage in the dark reached a plateau with an increase in the cultivation temperature earlier than with maintaining the initial conditions of the experiment. Whereas, in the light without changing the temperature regime, the percentage of germination was minimal and changed slightly, and after the transfer of already swollen seeds to conditions of 26°C and light, the germination process noticeably slowed down compared to the variant of the experiment in the darkness. This indicates that light had a significant effect on the growth rate of the embryo, both during and after the swelling of seeds. Although a heterogeneity of seeds in sensitivity to light occurred, for most of the seeds used in the experiment, light markedly increased the germination time.
Seed germination response to light depends on many conditions: its intensity and duration of exposure, temperature, atmospheric gas composition, osmotic factor, as well as on the water content and state of the seeds themselves. It can vary in different species of the same genus, or even in different varieties within the same species (Nikolaeva et al., 1999). The present study revealed the phenomenon of photosensitivity of the C. dayanum seed germination process. At the moment, we do not know how often it is manifested in this species. It is possible that this phenomenon is typical for the rare white-flowered form or only for a part of seeds including those used in the study. However, it should be emphasized that literature data and our results show that C. dayanum obtain intraspecific variability not only in the shape and size of the embryo inside the seed, but also in sensitivity to environmental factors in the course of seed germination.
Список литературы
Andronova E.V., Kulikov P.V., Philippov E.G., Vasilyeva V.E., Batygina T.B. 2009. Problems and Perspectives of in vitro seed propagation in orchids of temperate zone. In: Embryology of flowering plants. Terminology and concepts. Vol. 3. Reproductive systems. Enfield USA, Plymouth UK. P. 367–375, 567.
Arditti J. 1967. Factors affecting the germination of orchid seeds. – The Botanical Review. 33 (1): 1–97.
Arditti J. 1982. Tropical orchids (epiphytic and terrestrial). In: Orchid Biology: Reiews and Perspectives. Vol II. Ithaca, London. P. 273–278.
Averyanov L.V., Averyanova A.L., Nguyen K.S., Orlov N.L., Maisak T.V., Nguyen H.T. 2018. New and rare orchid species (Orchidaceae) in the flora of Cambodia and Laos. – Novitates Syst. Pl. Vasc. 49: 24–41. https://doi.org/10.31111/novitates/2018.49.24
Chang C., Ying C.C., Yen H.F. 2005. Protocorm or rhizome? The morphology of seed germination in Cymbidium dayanum Reichb. – Bot. Bull. Acad. Sin. 46: 71–74.
Cherevchenko T.M., Lavrentyeva A.N., Ivannikov R.V. 2008. Biotechnology of tropical and subtropical plants in vitro. Kyiv. 560 p. (In Russ.).
De L.C. Varietal Wealth in Orchids. 2020. – Biotica Research Today. 2 (4): 40–46.
Fast G. 1982. European Terrestial Orchids (symbiotic and asymbiotic methods). – In: Orchid Biology: Reiews and Perspectives. Vol. II. Ithaca, London. P. 309–326.
Hartini S., Wawangningrum H. 2019. Orchids from Mount Sago Nature Reserve, West Sumatera. – In: Proceedings SATREPS Conference. 1: 137–145.
Harvais G. 1973. Growth requirements and development of Cypripedium reginae in axenic culture. – Can. J. Bot. 51 (2): 327–332.
Johnson T.R., Kane M.E. 2012. Effects of temperature and light on germination and early seedling development of the pine pink orchid (Bletia purpurea). – Plant Species Biology. 27: 174–179. https://doi.org/10.1111/j.1442-1984.2011.00347.x
Kauth P.J., Dutra D., Johnson T.R., Stewart S.L., Kane M.E. Vendrame W. 2008. Techniques and applications of in vitro orchid seed germination. – In: Floriculture, Ornamental and Plant Biotechnology. V. 375–391.
Kolomeitseva G.L., Antipina V.A., Shirokov A.I., Khomutovskiy M.I., Babosha A.V., Riabchenko A.S. 2012. Orchid seeds: development, structure, germination. Moscow. 352 p. (In Russ.).
Kumar S., Singh P.D., Devi H.S., Thongam B., Somkuwar B.G., Thorat S.S. 2018. Cymbidium dayanum and Cymbidium sinense (Orchidaceae): two new additions to the orchid wealth of Manipur, India. – Richardiana. 2: 82–87.
Mahendran G., Muniappan V., Ashwini M., Muthukumar T., Bai V.N. 2013. Asymbiotic seed germination of Cymbidium bicolor Lindl. (Orchidaceae) and the influence of mycorrhizal fungus on seedling development. – Acta physiologiae plantarum. 35 (3): 829–840. https://doi.org/10.1007/s11738-012-1127-3
Matsuda Y., Sugiura N. 2019. Specialized pollination by honeybees in Cymbidium dayanum, a fall–winter flowering orchid. – Plant Species Biol. 34 (1): 19–26. https://doi.org/10.1111/1442-1984.12231
Matsui T., Kawai K., Samata Y. 1970. The effects of' `N-benzylaminopurine and α-naphthaleneacetic acid on organogénesis in Cymbidium. – Bulletin of the Faculty of Agriculture. Tamagawa University. 10: 99–106.
Nahar S.J., Shimasaki K., Haque S.M. 2013. In vitro growth and development of Cymbidium orchid. – In: 11th Asia Pacific Orchid Conference. P. 171–176.
Nikolaeva M.G., Lyanguzova I.V., Pozdova L.M. 1999. Biologiya semyan [The biology of seeds]. St. Petersburg. 231 p. (In Russ.)
Nongdam P., Chongtham N. 2012. In vitro seed germination and mass propagation of Cymbidium dayanum Reichb.: An important ornamental orchid of North-East India. – Trends in Horticultural Research. 2 (2): 28–37. https://doi.org/10.3923/thr.2012.28.37
Paek K.-Y., Murthy H.N. 2002. Temperate oriental Cymbidium species. Orchid Biology: Reviews and Perspectives. Vol. VIII. Dordrecht, Boston, London. P. 235–286.
Pozdova L.M., Titova G.E., Butuzova O.G. 2008. Seed germination in Fritillaria pallidiflora (Liliaceae) under gibberellins and kinetin influence. – Rastitelnye resursy. 4: 30–42 (In Russ.).
Prasad R.N., Mitra G.C. 1975. Nutrient requirements for germination of seeds and development of protocorms and seedlings of Cymbidium in aseptic cultures. – Indian Journal of Experimental Biology. 13 (2): 123–126.
Pratt L.W., Bio K.F. 2012. New plant records from Hawaii Island. – Bishop Museum Occasional Papers. 113: 75–80.
Pridgeon A.M., Cribb P.J., Chase M.W., Rasmussen F.N. 1999. Genera Orchidacearum. Vol. 1. Apostasioideae and Cypripedioideae. Oxford. 240 p.
Rasmussen H.N. 1995. Terrestrial orchids: from seed to mycotrophic plant. Cambridge. 460 p.
Rasmussen H.N., Dixon K.W., Jersáková J., Těšitelova T. 2015. Germination and seedling establishment in orchids: a complex of requirements. – Annals of Botany. 116: 391–402. https://doi.org/10.1093/aob/mcv087
Tawaro S., Suraninpong P., Chanprame S. 2008. Germination and regeneration of Cymbidium findlaysonianum Lindl. on a medium supplemented with some organic sources. – Walailak Journal of Science and Technology (WJST). 5 (2): 125–135.
Veyret Y. 1965. Embryogénie compare et blastogénie chez les Orchidaceae. – Monandrae. Paris. P. 1–106.
Veyret Y. 1974. Development of the embryo and the young seedling stages of orchids. – In: The orchids scientific studies. New York etc. P. 223–265.
Vinogradova T.N., Andronova E.V. 2002. Development of orchid seeds and seedlings. – In: Orchid biology: Reviews and Perspectives. Vol. VIII. Dordrecht Boston, London. P. 167–234.
Voges J.G., Rafael Fonseca Benevenuto R.F., Fritsche Y., Guerra M.P. 2014. Protocorm development of Epidendrum fulgens (Orchidaceae) in response to different saline formulations and culture conditions. – Acta Scientiarum. Biological Sciences. 36 (3): 287–292.
Yuanhua L., Qingyun L., Rao M., Yeyuan C. 2008. Asymbiotic germination and low–temperature in vitro conservation of Cymbidium dayanum. – Agricultural Science and Technology. 36 (19): 8068–8069, 8119.
Yakovlev M.S., Zhukova G.Ya. 1973. Angiosperms with green and colourless embryo (chloro- and leucoembryophytes). Leningrad. 101 p. (In Russ.).
Дополнительные материалы отсутствуют.
Инструменты
Ботанический журнал