Физика металлов и металловедение, 2023, T. 124, № 6, стр. 500-516
Систематическое описание термодинамических, упругих и механических свойств бинарных ОЦК сплавов на основе Zr из первых принципов
Е. А. Смирнова a, А. В. Пономарева a, Д. А. Конов a, М. П. Белов a, *
a НИТУ МИСИС
119049 Москва, Ленинский просп., д. 4, стр. 1, Россия
* E-mail: m.belov@misis.ru
Поступила в редакцию 27.03.2023
После доработки 24.04.2023
Принята к публикации 29.04.2023
- EDN: WWEYKL
- DOI: 10.31857/S0015323023600491
Аннотация
Эффекты влияния растворения 3d-, 4d- и 5d-металлов, а также Al, In и Sn в ОЦК-решетке Zr были исследованы в рамках теории функционала электронной плотности. С использованием метода EMTO-CPA были рассчитаны параметры решетки, энтальпии смешения, монокристаллические упругие константы С11, С12, С44 и C ', поликристаллические модули упругости E, G, а также характеристики пластичности разупорядоченных ОЦК-сплавов на основе Zr в широком концентрационном диапазоне до 50 ат. %. Методом PAW-SQS проведено исследование эффектов сплавления на указанные свойства ОЦК-сплавов Zr–X, где X – серии 4d-элементов Nb, Mo, Tc, Ru, Rh и 5d элементов Ta, W, Re, Os, Ir для концентрационных срезов 6.25, 25 и 50 ат. %. Проведен анализ концентрационных и периодических зависимостей свойств сплавов, их стабильности.
ВВЕДЕНИЕ
По своим физическим свойствам сплавы на основе Zr похожи на сплавы Ti [1, 2], при этом циркониевым сплавам уделено сравнительно мало внимания в научной литературе. Однако в последнее время интерес к сплавам, содержащим цирконий, возвращается [3–8]. Особенно перспективно сплавы на основе циркония выглядят с точки зрения их использования в качестве биоматериалов [9]. Zr и его сплавы обладают отличной биосовместимостью, хорошей коррозионной стойкостью и благоприятными механическими свойствами [10–16]. Одно из преимуществ Zr по сравнению с Ti – более низкая магнитная восприимчивость, что позволяет циркониевым имплантам меньше влиять на показания МРТ [17]. Исследования свойств сплавов циркония с переходными металлами Zr–Nb [18] и Zr–Mo [19] показывают, что при небольших концентрациях легирующего элемента происходит снижение магнитной восприимчивости, что, однако, сопровождается ухудшением механических характеристик. Важным преимуществом циркония является то, что он препятствует образованию фосфата кальция на импланте, и таким образом позволяет избежать повторного перелома кости во время операции удаления импланта [20].
Цирконий хорошо известен тем, что не поглощает или почти не поглощает нейтроны, поэтому является незаменимым компонентом при производстве ядерной энергии, где используется как внутреннее покрытие для реакторов и топливных ячеек. Поскольку здесь существует проблема окисления водяным паром, поглощения азота и водорода [21], то ее пытаются решить добавлением легирующих элементов, таких как Sn и переходных металлов Nb, Fe, Cr, Ni [22, 23].
Непосредственно бинарная система Ti–Zr также представляет интерес, поскольку металлы имеют неограниченную растворимость друг в друге и механические характеристики возрастают при увеличении концентрации одного в другом, достигая пика в районе 50 ат. % [24]. Кроме того, в данной системе наблюдается эффект памяти формы (ЭПФ). Это происходит благодаря наличию аллотропного превращения α (ГПУ) → β (ОЦК)-фаз чистого циркония при T = 1135 К. Температура фазового перехода зависит от легирующего элемента и его концентрации, т.е. легирующие элементы могут быть разделены на стабилизирующие или дестабилизирующие кубическую структуру циркония. Так, например, в работе [25] показано, что в сплаве Ti50Zr50 переход в мартенситную (гексагональную) фазу происходит при температуре 813 К и в кубическую фазу при 871 К, а в сплаве Ti70Zr30 при 913 и 933 К соответственно.
В работе [26] был обнаружен ЭПФ в сплаве (Ti–Zr)–1.5Mo–2Sn, где возврат к оригинальной форме изделия происходил при нагреве до 500 К. Данный ЭПФ связан с фазовым превращением из гексагональной метастабильной ω-фазы в кубическую. Формирование ω-фазы наблюдается во всех сплавах систем цирконий–переходной металл, в которых высокотемпературная ОЦК-фаза может быть сохранена в метастабильном состоянии при низких температурах [27]. Также она может быть получена и в чистом цирконии при ударных сжатиях вдоль направлений [0001] и [1–210] [28].
В данной работе мы не сосредотачиваемся на исследовании свойств циркониевых сплавов в применении к конкретной области использования, а даем общую картину разнообразия теоретически предсказанных термодинамических, упругих и механических свойств бинарных сплавов на основе ОЦК-циркония в широком интервале концентраций легирующего элемента от 0 до 50 ат. %, предсказываем их термодинамическую и механическую стабильность. В качестве легирующих элементов выбраны переходные 3d-, 4d- и 5d-металлы, а также некоторые р-элементы, такие как Al, In, Sn.
ДЕТАЛИ ВЫЧИСЛЕНИЙ
Расчеты электронной структуры и полной энергии проводили для температуры T = 0 K в рамках метода точных muffin‑tin орбиталей (EMTO) в сочетании с приближением когерентного потенциала (CPA) для моделирования беспорядка замещения [29, 30], а также с использованием метода проекционно‑присоединенных плоских волн (PAW) [31, 32], реализованный в пакете VASP [33–35]. Полную плотность заряда (FCD) [36] представляли одноцентровым разложением волновых функций электронов по сферическим гармоникам с орбитальными моментами lmax до 8. Для учета обменно‑корреляционных взаимодействий использовали приближение обобщенного градиента (GGA) [37]. Интегрирование в обратном пространстве проводили по сетке 37 × 37 × 37 k‑точек. Интегрирование энергии проводили в комплексной плоскости с использованием полуэллиптического контура, состоящего из 24 энергетических точек. Расчеты проводили для базисного набора, включающего валентные spdf‑орбитали. Спин‑поляризованные расчеты проводили для сплавов циркония с хромом, железом, марганцем, кобальтом и никелем. Упругие константы рассчитывали по деформационным зависимостям полных энергий. Для расчета модулей упругости поликристаллов использовали усреднение Хилла констант упругости монокристаллов.
Кристаллы кубической симметрии имеют три независимые упругие постоянные второго порядка: C11, C12 и C44, для которых критерий механической устойчивости задается соотношениями: C44 > 0, C11–C12 = 2C ' > 0, C11 + 2C12 > 0 [38].
Атомный беспорядок сплавов замещения моделировали в PAW с использованием техники специальных квазислучайных структур (SQS) [39], ОЦК‑структуру моделировали с помощью 128-атомной суперячейки. Энергия обрезания плоских волн была выбрана равной 450 эВ. Интегрирование зоны Бриллюэна выполнены с использованием набора 4 × 4 × 4k‑точек.
Метод EMTO был выбран в качестве высокопроизводительного инструмента расчета параметров решетки, энтальпий смешения и упругих и механических характеристик сплавов с 3d-, 4d-, 5d-переходными металлами, а также Al, Ga, Ge, In, Sn во всем диапазоне концентраций 0–50 ат. %. Метод PAW‑SQS более точный, но трудозатратный, был использован для расчета указанных характеристик лишь для серии 4d элементов Nb, Mo, Tc, Ru, Rh и 5d элементов Ta, W, Re, Os, Ir при трех концентрациях легирующего элемента 6.25, 25 и 50 ат. %.
Подробная методика расчетов термодинамических и упругих свойств для ОЦК-сплавов в методе EMTO‑CPA и PAW‑SQS приведена в работе [40].
РЕЗУЛЬТАТЫ
Термодинамические свойства
Концентрационные зависимости параметров решетки всех рассматриваемых систем, посчитанные методом EMTO представлены на рис. 1.
Рис. 1.
Рассчитанные методом EMTO-CPA параметры решетки ОЦК сплавов Zr–X, где X: a – 3d и Al, б – 4d и In, Sn, в – 5d-элементы.
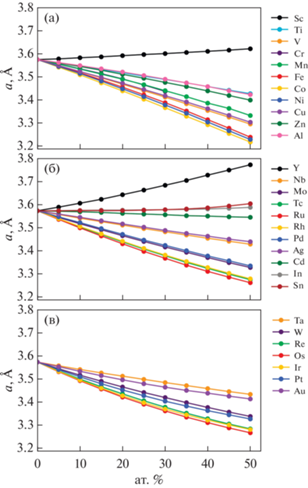
Можно видеть, что параметр решетки увеличивается только для сплавов Zr–Sc и Zr–Y, причем наибольшие значения достигаются при легировании иттрием. При добавлении p‑элементов, таких как In и Sn, параметр решетки незначительно (менее 1%) увеличивается, тогда как при легировании кадмием происходит малозаметное (~0.5%) уменьшение. Для остальных рассматриваемых элементов наблюдается уменьшение параметра решетки.
В сплавах циркония с 3d-металлами (рис. 1а) наблюдается уменьшение параметра решетки. Добавление титана незначительно уменьшает равновесный объем сплава, тогда как легирование ванадием приводит к более существенному снижению параметра решетки. Сплавы циркония с Cr, Mn, Fe, Co и Ni рассматривали с учетом магнитных взаимодействий в системе. Легирование хромом существенно повышает магнитный момент в системе, однако при концентрации ~35 ат. % Cr происходит магнитный переход, что приводит к резкому изменению свойств сплава. Поэтому в данной работе рассматривали сплавы до 30 ат. % Cr. При добавлении Mn, Fe и Co магнитный момент в сплавах монотонно увеличивается, что приводит к положительным отклонениям от закона Вегарда.
Максимальное значение магнитного момента достигается в сплаве Zr–50% Mn–1.14μБ. Для сплавов с кобальтом магнитный момент достигает 0.6μБ, и отклонение от линейного закона минимально. В сплавах циркония с никелем магнитный момент практически равен нулю. В ряду 3d-элементов максимальное снижение можно видеть в сплавах Zr–Co, тогда как значения параметра решетки для Zr–Fe и Zr–Ni лежат выше. Для элементов 4 периода вследствие магнитных взаимодействий в сплавах наблюдается смещение минимума параметра решетки к элементам с больше чем наполовину заполненной d‑зоной. Для элементов конца периода Cu и Zn концентрационные зависимости параметра решетки близки к V и Ti соответственно.
Рассмотрим изменения параметра решетки при добавлении 4d-металлов (рис. 1б). Для элементов начала и конца периода, таких как Nb и Ag, наблюдается уменьшение параметра решетки примерно на 4% при увеличении доли легирующих компонентов до 50%, тогда как для Mo и Pd это значение равно 8%. При легировании циркония элементами середины периода происходит значительное уменьшение параметра решетки. Минимальные значения наблюдаются в системе Zr–Ru, для сплавов Zr–Rh и Zr–Tc концентрационные зависимости параметров решетки очень близки и лежат чуть выше.
Для сплавов циркония с 5d-металлами (рис. 1в) параметр решетки монотонно уменьшается. При легировании Та параметр решетки снижается, в отличие от аналогичных сплавов с Ti [41], в которых добавление тантала приводит к незначительному увеличению равновесного объема сплава. Дальнейшее увеличение количества 5d‑электронов приводит к более существенному уменьшению параметра решетки. При легировании элементами середины периода наблюдается наиболее заметное уменьшение параметра решетки сплавов. Значения для сплавов Zr–Os являются минимальными для всех концентраций. Для металлов, находящихся ближе к концу периода, Pt и Au, изменения параметра решетки не столь значительны.
Можно отметить, что во всем концентрационном интервале при заполнении d-зоны параметр решетки сплавов с 3d-металлами значительно меньше, чем в сплавах с 4d- и 5d-элементами. При легировании элементами пятого и шестого периодов концентрационные зависимости параметров решетки очень похожи, так интервал изменения параметра решетки в ряду Nb–Ag практически совпадает с интервалом для ряда Ta–Au. В отличие от аналогичных сплавов с Ti [41], в циркониевых сплавах равновесный объем снижается существенней. Для сплавов циркония с элементами четвертого периода максимальное изменение достигает 10%, тогда как для титановых сплавов максимум около 8%.
Сравнение полученных результатов с имеющимися экспериментальными данными, а также значениями, полученными методом PAW‑SQS для некоторых систем, представлено на рис. П1 приложения. Рассчитанный параметр решетки меньше экспериментального, но результаты моделирования правильно воспроизводят концентрационные изменения, и отличие не превышает 1%.
На рис. 2 показаны рассчитанные значения энтальпии смешения ∆H (1) исследуемых сплавов.
(1)
$\Delta H = {{E}_{{{\text{Z}}{{{\text{r}}}_{{{\text{1}} - c}}}{{{\text{X}}}_{c}}}}} - (1 - c){{E}_{{{\text{Zr}}}}} - c{{E}_{{\text{X}}}},$Рис. 2.
Рассчитанные методом EMTO-CPA энтальпии смешения ОЦК-сплавов Zr–X, где X: a – 3d и Al, б – 4d и In, Sn, в – 5d-элементы.
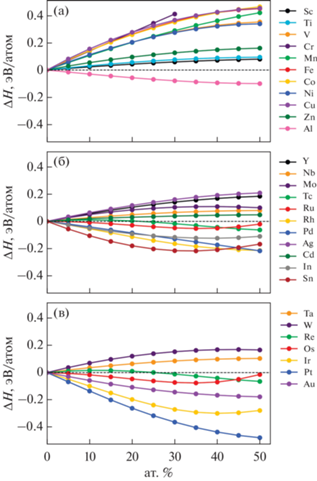
Значения ΔH > 0 (<0) свидетельствуют о склонности к фазовому расслоению (образованию твердого раствора). Энтальпии смешения рассчитывали относительно чистых элементов в основном состоянии: ферромагнитного (ФМ) ОЦК-Fe, ФМ ГЦК-Ni, ФМ ГПУ-Co, ОЦК-Cr в немагнитном (НМ) состоянии, ФМ α‑Mn, НМ ГЦК-Al, Cu, Ag, Au, Ir, Pt, Pd, Rh, ОЦК Mo, V, W, Nb, Ta, ГПУ Zn, Cd, Hf, Ti, Y, Sc, Re, Ru, Tc, орторомбический In, Sn в структуре алмаза.
Сплавы циркония с 3d-металлами демонстрируют положительную энтальпию смешения во всем концентрационном интервале (рис. 2a). Энтальпия смешения максимальна для сплавов с Cu, Fe и Co и достигает 0.45 эВ/атом. Сплав с алюминием является термодинамически устойчивым.
В ряду 4d-металлов наиболее термодинамически стабильным является сплав Zr–Rh (рис. 2б). Элементы начала периода Y, Nb, Mo, так же, как и элементы с практически полностью заполненной d‑зоной Ag, Cd, имеют положительную энтальпию смешения. При небольших концентрациях технеция ОЦК-сплав Zr–Tc нестабилен, однако увеличение доли легирующего компонента выше 20 ат. % приводит к стабилизации сплава. Сплавы циркония с рутением, родием и палладием устойчивы на всем концентрационном интервале. Увеличение количества p-электронов на внешней оболочке способствует стабилизации сплава (Zr–In, Zr–Sn).
Для сплавов с 5d-элементами (рис. 2в), энтальпия смешения минимальна в системе Zr–Pt. Сплавы Zr–Ir, Zr–Os, Zr–Au также термодинамически устойчивы во всем диапазоне концентраций. В сплавах Zr–Re увеличение содержания Re более 25 ат. % приводит к стабилизации сплава. В сплавах с Ta и W энтальпия смешения положительна при всех концентрациях легирующего элемента.
По сравнению с титановыми сплавами, соответствующие сплавы циркония демонстрируют меньшую термодинамическую стабильность. Все сплавы циркония с 3d-металлами неустойчивы, тогда как в системах Ti–Co, Ti–Ni, Ti–Zn, Ti–Fe и Ti–Mn наблюдалась отрицательная энтальпия смешения [41]. При сравнении сплавов титана и циркония с 4d-элементами не видно столь значительных отличий, но значения энтальпии смешения для титановых сплавов в среднем на 0.1 эВ/атом меньше. При легировании 5d-металлами можно отметить основное отличие при рассмотрении титановых и циркониевых сплавов c рением и вольфрамом, которые демонстрируют разную термодинамическую стабильность.
Упругие свойства
Концентрационная зависимость объемных модулей сплавов Zr–X представлена на рис. 3.
Рис. 3.
Рассчитанные методом EMTO-CPA значения объемного модуля ОЦК сплавов Zr–X, где X: a – 3d и Al, б – 4d и In, Sn, в – 5d-элементы.
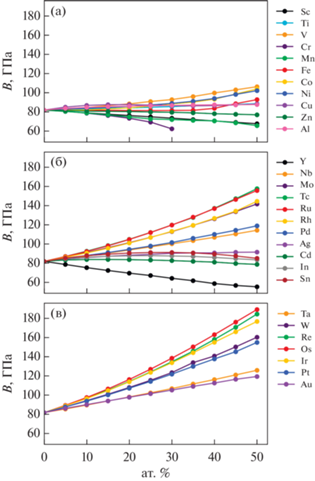
При добавлении Y или Sc объемный модуль сплавов существенно уменьшается, что соответствует значительному увеличению параметра решетки в этих сплавах. Можно видеть, что для сплавов с элементами начала и конца периода (Cu, Zn, Cd) так же, как и для сплавов с p‑элементами (Al, In Sn) концентрационные изменения объемного модуля очень незначительные и не превышают 10%.
При легировании титаном объемный модуль незначительно увеличивается (рис. 3a). Для сплава Zr–V значение B монотонно растет и достигает максимального значения 105 ГПа в ряду 3d-элементов. Для сплавов с Cr, Mn, Fe, Co и Ni существенное влияние на свойства оказывает магнитное взаимодействие. Объемный модуль Zr–Cr и Zr–Mn сплавов снижается, причем линия для Zr–Cr лежит даже ниже линии для сплава Zr–Sc. Для сплавов циркония с железом значение объемного модуля практически не меняется при увеличении концентрации до 35%, дальнейшее добавление легирующего элемента приводит к росту объемного модуля. Для сплавов Zr–Co и Zr–Ni наблюдается увеличение объемного модуля, причем для системы Zr–Co нелинейность зависимости выражена сильнее, что можно объяснить увеличением магнитного момента в системе. Можно предположить, что столь нелинейное поведение объемного модуля в ОЦК-циркониевых магнитных сплавах получается в результате конкуренции различных вкладов – магнитного и размерного факторов. При легировании элементами середины пятого и шестого периодов (рис. 3б, 3в) объемный модуль монотонно увеличивается. Наиболее быстрый рост происходит в имеющих сходную электронную структуру Zr–Os и Zr–Ru сплавах. В сплавах с 5d-металлами объемный модуль меняется наиболее значительно при заполнении d‑зоны. Наибольшее значение объемного модуля (190 ГПа) достигается при добавлении 50 ат. % осмия. Характер изменения объемного модуля при легировании циркония и титана 4d- и 5d-металлами очень похож, но диапазон изменений в циркониевых сплавах меньше. Для сплавов циркония и титана с 3d-металлами существенные различия объясняются сильными магнитными взаимодействиями в Zr–X сплавах.
Для исследования механических свойств сплавов были рассчитаны упругие константы C ', С11, С12, С44, показывающие отклик материала на различные деформации. Кроме того, константа C ' удобна для оценки механической стабильности сплавов с кубической решеткой (условие механической стабильности: C ' > 0).
Концентрационные зависимости константы C' показаны на рис. 4. Можно видеть, что для ОЦК-сплавов Zr–Sc и Zr–Y константа C ' становится меньше нуля при доле легирующего компонента более 15 ат. %, что указывает на сильную механическую нестабильность сплавов с данными легирующими элементами.
Рис. 4.
Рассчитанные методом EMTO-CPA значения константы упругости С ' ОЦК сплавов Zr–X, где X: a – 3d и Al, б – 4d и In, Sn, в – 5d-элементы.
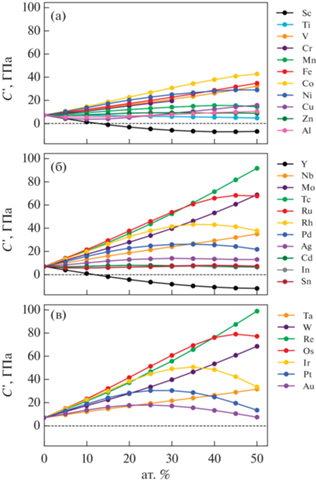
Рассмотрим изменение значений C ' при легировании 3d-металлам (рис. 4a). В сплавах с титаном значение C ' незначительно уменьшается. При легировании V, Cr, Fe и Co наблюдается практически линейный рост C ', максимальное значение достигается в сплаве Zr–Co – 48 ГПа. При легировании Mn и Ni значения C ' монотонно возрастают примерно до 40 ат. % и выходят на плато при дальнейшем увеличении концентрации, однако значения для сплавов Zr–Mn существенно ниже (28 и 14 ГПа соответственно). В сплавах Zr–Cu концентрационная зависимость C` имеет минимум на 15 ат. %, но общее изменение значений невелико. Аналогичная картина наблюдается в сплавах с Al.
На рис. 4б можно видеть, что значение C ' монотонно растет при добавлении Nb, Mo и Tc, наибольшее значение ~91 ГПа соответствует сплаву Zr–50% Tc. При дальнейшем увеличении количества d‑электронов концентрационная зависимость C ' принимает вид кривой с максимумом при плавном уменьшении максимальных значений. Для сплавов Zr–Ru максимум достигается при легировании 45 ат. %, тогда как для Zr–Cd он смещается на концентрацию 20 ат. %. При увеличении количества p‑электронов (In, Sn) значения C ' очень слабо возрастают (примерно на 2 ГПа).
При легировании циркония 5d-металлами наблюдается похожая картина (рис. 4в). При добавлении элементов, в которых d-зона заполнена меньше, чем наполовину, значения C ' линейно возрастают при повышении концентрации. При дальнейшем увеличении количества d-электронов так же, как и в случае 4d-элементов, видна нелинейная зависимость с максимумом, однако в этом случае максимум гораздо более выражен.
Концентрационные зависимости констант С11 и С12 показаны на рис. 5. Можно видеть, что характер изменения С11 (рис. 5б, 5в) для 4d- и 5d-элементов очень похож на соответствующие зависимости C '. Однако в случае C11 зависимости, имеющие максимум, существенно сглажены (Zr–Ir, Zr–Pt, Zr–Au) или выходят на плато (Zr–Ru, Zr–Rh, Zr–Pd, Zr–Ag). Максимальное значение 316 ГПа достигается для Zr–50%Re. Значительное увеличение параметров решетки для Zr–Y и Zr–Sc приводят к уменьшению С11. Для элементов конца периода и p‑элементов (Zn, Cd, Al, In, Sn) изменения С11 в зависимости от концентрации легирующего компонента очень малы и не превышают 10 ГПа. Для сплавов Zr–Cr, Zr–Mn значение упругой константы С11 практически не меняется, что связано с уменьшением объемного модуля сплава. При легировании 3d-металлами, имеющими более чем наполовину заполненную d‑зону (Fe, Co, Ni), происходит практически линейный рост значений С11.
Рис. 5.
Рассчитанные методом EMTO-CPA значения констант упругости С11 (a–в) и С12 (г–е) ОЦК-сплавов Zr–X, где X: a, г – 3d и Al, б, д – 4d и In, Sn, в, е – 5d-элементы.
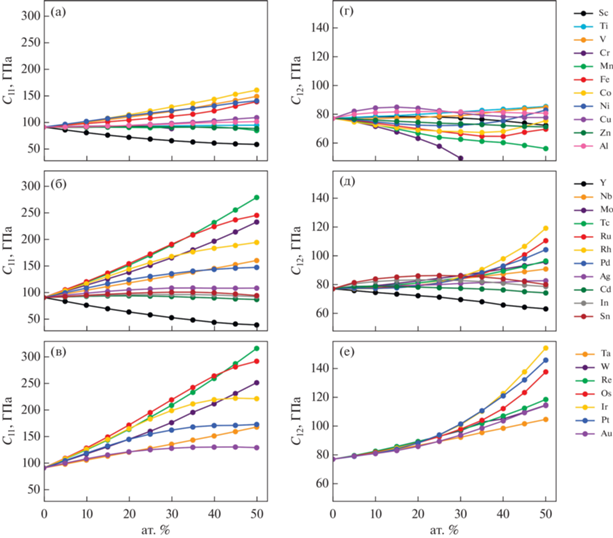
Рассчитанные значения С12 приведены на рис. 5г–5е. При добавлении Ti и V C12 монотонно увеличивается, однако суммарное изменение значений не превышает 10 ГПа. Значение С12 существенно уменьшается для сплавов Zr–Cr и Zr–Mn. При добавлении Fe, Co и Ni кривая имеет минимум, который смещается от 35 ат. % для Fe до 20 ат. % для Ni. Для других элементов четвертого периода С12 меняется очень слабо.
Для сплавов с 4d-металлами характерно незначительное изменение (менее 10%) значения С12 для элементов конца периода и p‑элементов (Ag, Zr, Cd, In, Sn). При легировании элементами середины периода (Nb, Mo, Tc, Ru, Rh, Pd) наблюдается монотонное увеличение значений С12. Наибольший рост достигается при легировании Rh, Ru и Pd. В ряду 5d-металлов значения С12 для всех рассматриваемых сплавов монотонно растут. Максимальное значение 157 ГПа достигается для сплава Zr–50 ат. % Ir.
Рассчитанные значения упругой константы С44 показаны на рис. 6. Можно видеть, что при легировании 3d-металлами, такими как Sc, Ti, Mn, Cr, Ni, изменение константы С44 не превышает 10%. Добавление ванадия и железа приводит к монотонному уменьшению С44, тогда как при легировании кобальтом наблюдается нелинейная зависимость с минимумом при 25 ат. %. Из всех 3d-металлов наименьшее значение С44 имеет сплав Zr–Zn в интервале концентраций 0–45 ат. %. При увеличении содержания меди концентрационная зависимость имеет максимум на 20 ат. %. Аналогичная картина наблюдается для металлов с похожей электронной структурой (Ag, Au), однако там максимум смещается на 25 ат. %, но диапазон изменений уменьшается. Добавление алюминия приводит к монотонному росту константы С44.
Рис. 6.
Рассчитанные методом EMTO-CPA значения константы упругости С44 ОЦК-сплавов Zr–X, где X: a – 3d и Al, б – 4d и In, Sn, в – 5d-элементы.
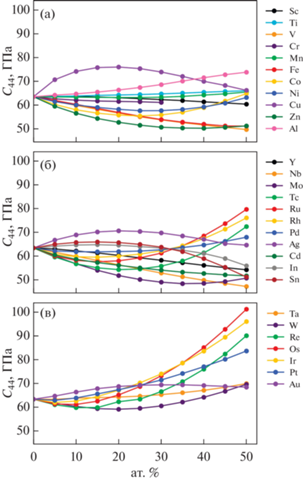
При легировании элементами середины пятого периода (Mo, Tc, Ru, Rh) наблюдается нелинейная зависимость, при этом минимум на кривой смещается с 35 ат. % для Mo до 10 ат. % для Rh. Похожую концентрационную зависимость демонстрируют элементы середины шестого периода (W, Re, Os, Ir), однако в этом случае минимум смещается с 20 ат. % для W и до 5 ат. % для Ir. Можно видеть, что минимальные значения С44 наблюдаются для сплавов Zr–Mo (Zr–W) практически во всем концентрационном интервале. При легировании p-элементами (In, Sn) концентрационная зависимость имеет максимум при 15 ат. %. Максимальный диапазон изменений соответствует сплавам с 5d-металлами.
В целом можно видеть, что циркониевые и титановые сплавы [41] с 4d- и 5d-металлами имеют похожие концентрационные зависимости упругих констант, однако сплавы с титаном достигают более высоких значений. Для сплавов с 3d-элементами различия в поведении упругих констант можно объяснить существенным магнитным взаимодействием в циркониевых сплавах, тогда как в титановых сплавах это взаимодействие очень мало.
Концентрационные зависимости модулей Юнга и модулей сдвига Zr–X сплавов представлены на рис. 7.
Рис. 7.
Рассчитанные методом EMTO‑CPA значения модуля сдвига G (a, б, в) и модуля Юнга E (г, д, е) ОЦК-сплавов Zr–X, где X: a, г – 3d и Al, б, д – 4d и In, Sn, в, е –5d-элементы.
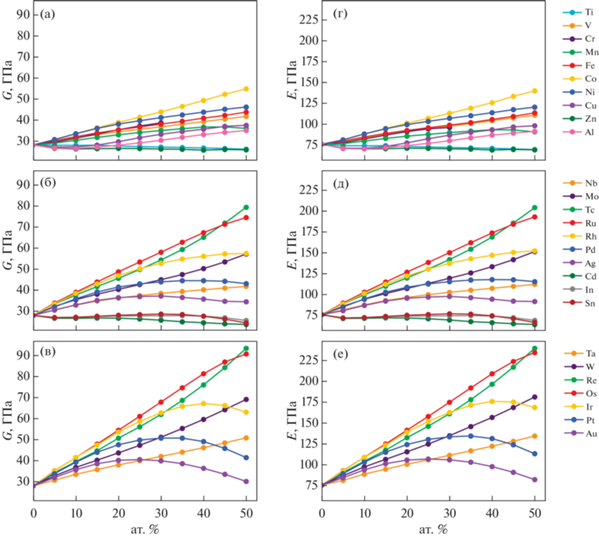
Можно видеть, что тенденции изменения модулей очень похожи. В сплавах циркония с титаном наблюдается монотонное уменьшение модулей, однако суммарное изменение составляет всего 10%. Концентрационные зависимости при легировании ванадием и железом линейно возрастают и очень близки. Добавление марганца до 35 ат. % приводит к возрастанию значений модулей и последующему незначительному падению при уменьшении доли циркония. Для сплавов с медью и алюминием концентрационные зависимости имеют вид кривой с минимумом около 10 ат. %. Максимальные значения в ряду 3d-металлов достигаются при увеличении содержания кобальта.
Рассмотрим изменения значений модулей при легировании 4d и 5d-металлами. По мере заполнения d‑зоны, значения модулей монотонно линейно возрастают при увеличении концентрации легирующего элемента. Скорость роста больше для сплавов с 5d-элементами. Диапазон изменения модуля Юнга от 78 до 240 ГПа, модуля сдвига от 27 до 92 ГПа. Для сплавов Zr–Ru и Zr–Os при CX = 50 ат. % значения очень близки к Zr–Tc и Zr–Re соответственно. При дальнейшем увеличении количества d‑электронов на внешнем уровне на концентрационных зависимостях модуля Юнга и модуля сдвига появляется максимум, который смещается в сторону уменьшения количества легирующего элемента. Для Zr–Ir наибольшее значение модуля Юнга достигается при 40 ат. %, для Zr–Pt при 30 ат. % и 25 ат. % для Zr–Au. Для металлов 4d-периода возникает похожая ситуация при добавлении Rh, Pd, Ag, однако максимумы смещаются в сторону больших концентраций (Zr–Pd, Zr–Ag) или практически выходят на плато как в случае Zr–Rh. При легировании p‑элементами (In, Sn) значения модуля Юнга и модуля сдвига незначительно (до 10%) уменьшаются.
Рассмотрим механические свойства циркониевых сплавов. Для оценки пластичности сплава были использованы три параметра: отношение G/B, коэффициент Пуассона ν и параметр упругой анизотропии Зенера Az = 2C44/(C11 – C12). При G/B < 0.5 и ν > 0.3 материал предполагается пластичным с металлическим типом связи [42, 43].
Можно видеть (рис. 8), что критерию G/B < 0.5 удовлетворяют все сплавы циркония с 3d-металлами, кроме сплавов с хромом, марганцем и кобальтом при концентрации легирующего компонента более 30 ат. % (для Cr 20 ат. %).
Рис. 8.
Рассчитанные методом EMTO‑CPA значения параметра G/B (a, б, в) и коэффициента Пуассона ν (г, д, е) ОЦК сплавов Zr–X, где X: a, г –3d и Al, б, д – 4d и In, Sn, в, е –5d-элементы.
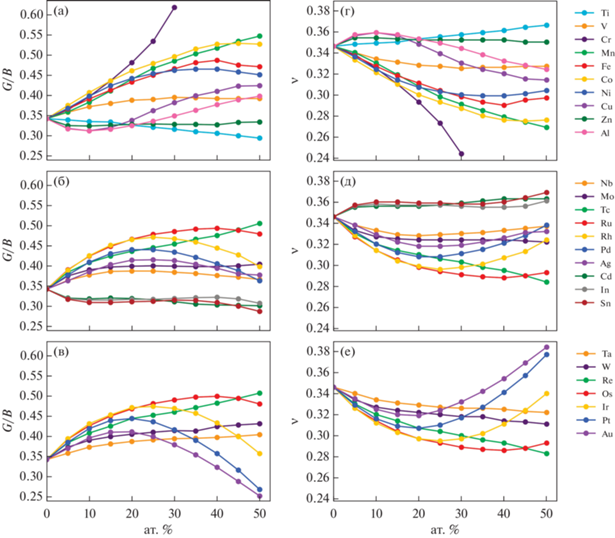
Коэффициент Пуассона в сплавах Zr–Fe, Zr–Co, Zr–Mn, Zr–Cr также становится меньше 0.3 при уменьшении содержания циркония, что свидетельствует о тенденции к повышению хрупкости данных сплавов. При добавлении никеля коэффициент ν очень близок к 0.3, что показывает повышение степени ковалентности связи в этих сплавах. Параметр анизотропии Az сплавов Zr–X уменьшается при увеличении доли X, где X – элементы середины периода до значений Az ~ ~ 1.5–2, однако для сплавов Zr–Mn остается достаточно высоким (~6). Для сплавов с 4d-металлами критерий G/B < 0.5 выполняется для всех композиций, за исключением Zr–50 ат. % Tc, где G/B = 0.505. При легировании элементам начала и середины периода параметр ν уменьшается и для сплавов Zr–Tc и Zr–Ru становится меньше 0.3 при концентрации 35 и 20 ат. % для Tc и Ru соответственно. При дальнейшем заполнении d‑зоны на концентрационной зависимости коэффициента Пуассона появляется минимум в районе 35 ат. % для сплавов с Rh, Pd, Ag. При легировании p‑элементами (In, Sn) сплавы становятся более пластичными, чем чистый цирконий. Рассматриваемые сплавы циркония с элементами шестого периода являются пластичными согласно критерию G/B < 0.5. Аналогично сплаву Zr–50 ат. % Tc, значение G/B слегка превышает 0.5 для сплава Zr–50 ат. % Re.
Для сплавов с Os, Ir, Pt, Au концентрационная зависимость параметра G/B имеет максимум. Для сплавов с высоким содержанием Ir наблюдается достаточно значительное уменьшение параметра G/B, связанное с резким падением значения упругой константы C ' и, соответственно, модуля сдвига. В целом зависимость коэффициента Пуассона хорошо коррелирует с параметром G/B.
Для элементов начала периода ν уменьшается с увеличением концентрации CX. При легировании элементами с наполовину заполненной d-зоной на кривой появляется минимум, примерно соответствующий максимуму на линии G/B. Для сплавов Zr–Tc, Zr–Ru (Zr–Re, Zr–Os) при уменьшении содержания циркония параметр G/B увеличивается и достигает практически 0.5, а коэффициент Пуассона ν становится меньше 0.3, что хорошо согласуется с рассчитанными высокими механическими характеристиками. Параметр анизотропии для сплавов с 4d- и 5d-металлами уменьшается при увеличении концентрации легирующего компонента, но при добавлении элементов с более чем наполовину заполненной d‑зоной начинает расти при концентрации CX более 30 ат. %. Наиболее существенный рост наблюдается для сплавов Zr–Pt и Zr–Au.
Можно видеть, что по сравнению с титановыми сплавами [41], сплавы циркония менее пластичны и имеют более высокую степень ковалентности связей.
Доступные литературные экспериментальные и теоретические данные по механическим характеристикам циркониевых сплавов приведены в табл. П1 приложения. Сравнение значений упругих констант и объемного модуля, полученных методом EMTO‑CPA, показывает хорошее согласие с экспериментальными данными для системы Zr–Nb [44] и Zr–Ti. Можно видеть, что правильно воспроизводятся все тенденции изменения модулей сплава Zr–Nb, а отличия в значениях B, С11 и С12 не превышают 4%. Также наблюдается хорошее согласие с теоретическими данными других авторов.
Таблица 1.
Параметры решетки и упругие свойства ОЦК Ti и Zr (PAW‑SQS)
Упругие свойства/элемент | C11, ГПа | C12, ГПа | C ', ГПа | C44, ГПа | B, ГПа | E, ГПа | G, ГПа | ν | Az | a, Å |
---|---|---|---|---|---|---|---|---|---|---|
Zr | 83 | 90 | –3 | 32 | 88 | 12 | 4 | 0.48 | –9.5 | 3.574 |
Ti [41] | 88 | 113 | –12 | 41 | 105 | –58 | –18 | 0.59 | –3.3 | 3.253 |
ВЕРИФИКАЦИЯ РЕЗУЛЬТАТОВ EMTO‑CPA С ИСПОЛЬЗОВАНИЕМ МЕТОДА PAW‑SQS
Расчеты методом PAW‑SQS проведены для сплавов с немагнитными элементами середины 5-го и 6-го периодов, которые максимально стабилизируют Zr–X-сплавы, и в расчетах EMTO-CPA имеются экстремумы на кривых механических характеристик.
Упругие свойства ОЦК-Zr, полученные с помощью PAW‑SQS, приведены в табл. 1. Условие C ' < 0 указывает на механическую нестабильность Zr в ОЦК-структуре при T = 0 K. Действительно, Zr при комнатной температуре имеет гексагональную плотноупакованную структуру, которая при T = 872°C аллотропно трансформируется в объемно‑центрированную кубическую структуру [45]. При сравнении с нашими результатами для чистого ОЦК-титана [41] видно, что цирконий имеет менее отрицательное значение C' и соответственно, положительные значения модулей Юнга E и сдвига G, но также нефизические характеристики анизотропии. Это качественно совпадает с результатами работы [46], в которой показано, что разница структурных энергий Ebcc‑hcp для Ti и Zr положительны, при этом значения для титана в ~1.3 раза выше, чем для циркония.
Как показано в работах [41, 46] на примере ОЦК-Ti сплавов с низким содержанием второго компонента, для которых наблюдается динамическая и механическая неустойчивость, оптимизация суперячеек с релаксацией атомных позиций приводит к структуре, далекой от ОЦК. В полученной релаксированной структуре почти все атомы в суперячейке выходят из идеальных ОЦК-позиций. При этом использование релаксированной ОЦК-суперячейки для расчета энтальпии смешения ΔH при T = 0K приводит к экзотермической реакции в растворе на основе Ti. В то же время эксперимент и AIMD расчеты при T ~ 1300 K предсказывают положительные значения ΔH [47]. Поэтому для малых концентраций второго элемента CX = 6.25 ат. % мы проводили расчеты упругих и термодинамических характеристик ОЦК Zr–X-сплавов на идеальных суперячейках. Ориентируясь на экспериментальные результаты работ [44, 48, 49], в которых показано, что ~20 ат. % V, Ta, Nb в титане и 30 ат. % Nb в цирконии являются критическими концентрациями для получения 100% ОЦК-твердого раствора, мы выполнили расчеты для концентраций CX = 25 и 50 ат. %. на идеальных и релаксированных ячейках, в которых оптимизацию проводили по объему и позициям атомов, что позволило оценить эффект статической релаксации на величины упругих характеристик. На рис. 9 и рис. 10 представлены термодинамические и упругие характеристики ОЦК Zr–X-сплавов при легировании Zr элементом X (4d: Nb, Mo, Tc, Ru, Rh; 5d: Ta, W, Re, Os, Ir) для концентрации второго компонента CX = 6.25, 25 и 50 ат. %, полученные на идеальной ячейке. Добавление 6.25 ат. % X к Zr увеличивает C11 для всех X, С12 при легировании 4d (5d)-примесей немного ниже (выше) значений чистого циркония, но величины меняются достаточно слабо. Кривая значений C ' имеет слабо выраженный максимум для элементов Ru (4d) и Os (5d), при этом все значения положительны, т.е. легирование циркония любым из изучаемых элементов приводит к выполнению условия механической стабильности C ' > 0. Отметим, что титановые сплавы с Nb, Ta, W имели отрицательные значения константы C ' [41]. Зависимости C44 имеют несимметричную параболическую форму с минимумами на элементах Tc (4d) и Re (5d), при этом все значения немного ниже, чем C44 чистого циркония.
Рис. 9.
Упругие константы Cij, параметры решетки a, энтальпии смешения ΔH ОЦК Zr–X-сплавов с 4d (зеленые линии) и 5d-элементами (красные линии), рассчитанные с помощью метода PAW-SQS на идеальной ячейке. Квадраты соответствуют концентрации второго компонента CX = 6.25 ат. %, круги – 25 ат. %, ромбы – 50 ат. %.
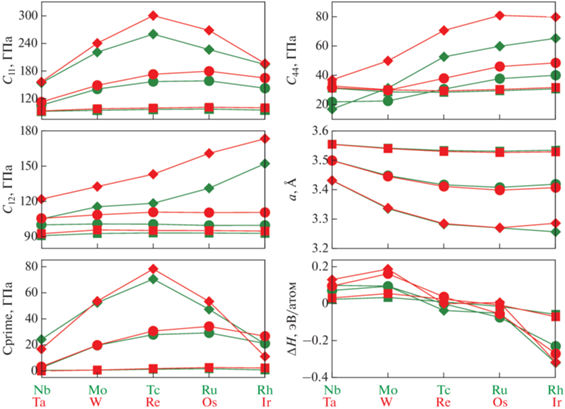
Рис. 10.
Упругие свойства (Hill) ОЦК Zr–X-сплавов с 4d (зеленые линии) и 5d-элементами (красные линии), рассчитанные с помощью метода PAW‑SQS на идеальной ячейке. Квадраты соответствуют концентрации второго компонента CX = 6.25 ат. %, круги –25 ат. %, ромбы – 50 ат. %.
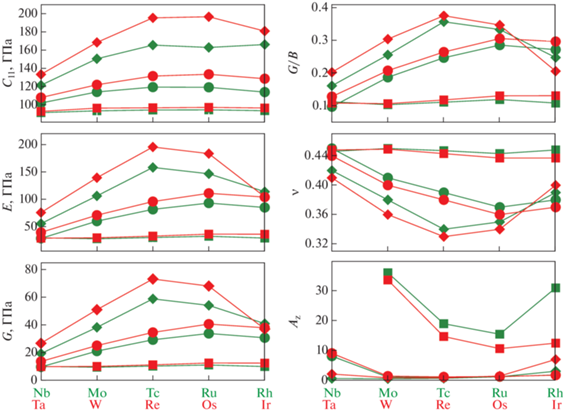
Значения энтальпии смешения (ΔH), определенные по отношению к распаду на чистые элементы в их основных состояниях, отражают в том числе и степень стабильности межатомных связей в структуре основного состояния элемента. В данном случае мы наблюдаем сходство поведения кривой ΔH и зависимостей энергии когезии элементов вдоль периодов [50]: максимум для W и самые низкие значения для сплава с Ir.
Значения объемных модулей B (рис. 10) меняются слабо в рядах рассматриваемых элементов, зависимости модуля сдвига G и модуля Юнга для этой концентрации имеют кривые с изломами при движении по d-ряду, но также меняются незначительно, максимальное изменение показывает модуль Юнга для сплавов с Os и Ir, достигая значения 36 ГПа, т. е. находится в области значений, необходимых для биомедицинских применений. Наблюдаются высокие значения коэффициента анизотропии Az из‑за близких к нулю C ' для этой концентрации X. Чрезвычайно низкие значения параметра G/B и, напротив, высокие значения коэффициента Пуассона ν свидетельствуют о пластичности материалов и металлическом характере атомной связи в Zr–X-сплавах при малых концентрациях X. Также низкие значения G/B рассматривают как индикатор очень высокой вязкости разрушения.
При увеличении концентрации X до 25 ат. % (рис. 9, 10) константы С11 значительно увеличиваются относительно CX = 6.25 ат. %, что при несущественном изменении константы С12 приводит к увеличению C ' и соответственно, стабилизации всех сплавов системы. Минимальное увеличение C ' испытывают сплавы с Nb и Ta, максимальное – с Ru и Os. Значения константы С44 продолжают уменьшаться для сплавов с Nb, Mo и W, для остальных сплавов увеличиваются относительно Zr и сплавов с CX = 6.25 ат. %. Значения энтальпии смешения становятся более отрицательными, за исключением Mo и W.
Значения модулей B, G, E, соотношения G/B также увеличиваются при увеличении концентрации второго компонента до 25 ат. % (рис. 10), модули Юнга E имеют максимумы, как и C11, на Ru и Os. Значения модуля Юнга E изменяются в диапазоне 30–90 ГПа, модуль сдвига G – 10–35 ГПа. Параметр G/B резко увеличивается, что свидетельствует об уменьшении пластичности, в то же время коэффициент Пуассона уменьшается через минимум на Os и Ru. Параметр анизотропии Az остается высоким только для Nb и Ta, для всех остальных элементов Az становится близким к 1–2.
Для концентрации второго элемента CX = = 25 ат. % было проведено исследование влияния релаксации ячейки на свойства сплавов (рис. П2 и П3). Показано, что оптимизация структуры сохраняет ОЦК-решетку почти всех элементов. Для Rh и Os наблюдаются локальные смещения некоторых примесных атомов, для сплава с Ir области таких смешений начинают объединяться в нелокальный структурный дефект.
Константа С11 релаксированных сплавов показывает рост при движении по d-ряду, С12 при этом уменьшается, что приводит к увеличению C', особенно для элементов начала и середины периода, и соответственно, увеличению механической стабильности системы (рис. П2). Константа С44 релаксированных ячеек выше значения чистого Zr и сплавов с концентрацией X = 6.25 ат. %. для всех элементов. После релаксации наблюдается увеличение значений E, G, G/B, также наблюдается уменьшение параметра анизотропии для Ta, Nb, уменьшения коэффициента Пуассона ν (рис. П3).
Одновременное увеличение С44, параметра G/B и уменьшение ν указывает на возрастание ковалентной составляющей атомной связи в этих сплавах, которая приводит к увеличению механических характеристик. Особенно ярко этот эффект проявляется для 4d-элементов начала периода. При этом для сплавов с Mo, Tc, Re параметры анизотропии Az = 1–1.1, это указывает на большую степень полярности ковалентной связи с этими элементами.
Экспериментальные данные для концентрации Zr–30Nb [41] (C11 = 128 ГПа, C12 = 89 ГПа, C44 = 35 ГПа, C ' = 20 ГПа, B = 102 ГПа, Az = 1.75) значительно ближе для релаксированной Zr–25Nb ячейки (C11 = 137 ГПа, C12 = 92 ГПа, C44 = 35 ГПа, C ' = 23 ГПа, B = 107 ГПа, Az = 1.6), чем для идеальной (C11 = 105 ГПа, C12 = 100 ГПа, C44 = 22 ГПа, C' = 2.7 ГПа, B = 102 ГПа, Az = 8.2). При увеличении концентрации второго компонента X до 50 ат. % (рис. 9, 10) значения C11 продолжают расти с максимальным увеличением для элементов середины периода Re и Tc. Константы C12 на данной концентрации показывают линейный рост от Ta к Ir (от Nb к Rh), что приводит к появлению ярко выраженного максимума C ' в районе Re (Tc) и сильного понижения C' для Zr–50Ir сплава. Константа С44 увеличивается для всех элементов, кроме Nb, максимальные значения наблюдаются для Rh и Ir. Энтальпия смешения слабо меняется относительно 25 ат. %, показывая максимальные положительные значения для W (Mo) и минимальные отрицательные для Ir (Rh).
При увеличении концентрации до 50 ат. % наблюдается дальнейшее увеличение объемного модуля B, максимальное для сплавов с Re и Os ~ ~ 200 ГПа, модуль сдвига G и модуль Юнга E, параметр G/B также продолжают расти, достигая, соответственно ~80 ГПа, ~200 ГПа, ~0.4 для Zr–50Re сплава. Параметры ν и Az уменьшаются для всех элементов, за исключением Ir и Rh, для которых наблюдается уменьшение значений G, E, G/B и увеличение ν и Az относительного данных, соответствующих 25 ат. %. Это является следствием резкого уменьшения упругих констант в Zr–50Ir и Zr–50Rh-сплавах.
Для CX = 50 ат. % также проведена оценка влияния релаксации на механические и термодинамические свойства Zr–50X-сплавов, которая, как и для CX = 25 ат. %, показала увеличение механических характеристик и стабильности Zr–X-сплавов. Сильный эффект релаксации наблюдается для сплавов с Rh и Ir, для которых обнаружено значительное увеличение констант C11, уменьшение С12 и, как следствие, существенное увеличение упругой константы C' относительно расчетов для идеальной ячейки (рис. П2). Константа C44 для сплавов с этими элементами уменьшается при оптимизации структуры. Однако нужно отметить, что релаксация в Zr–50Ir и Zr–50Rh-сплавах, особенно в Zr–50Ir, приводит к потере порядка ОЦК-структуры и хаотичному размещению атомов в ячейке (рис. 11). Это свидетельствует о маловероятном сохранении твердого раствора при данной концентрации, что подтверждает наличие целого ряда интерметаллических фаз в системе Ir–Zr [51]. При этом влияние на поликристаллические модули E и G оказывается для Ir и Rh не самое большое; как и при концентрации 25 ат. %, максимальное увеличение E, G, G/B наблюдается для сплавов с элементами начала периода (рис. П3).
Рис. 11.
Равновесные положения атомов в релаксированных суперячейках Zr–X-сплавов (слева направо) Zr–50Ta, Zr‒50W, Zr–50Re, Zr–50Os, Zr–50Ir, полученных методом статической релаксации.
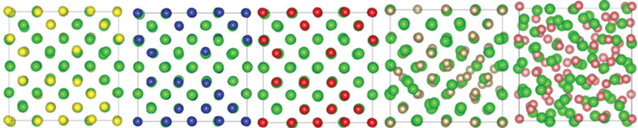
Экспериментальные данные для Zr–50Nb (C11 = 156 ГПа, C12 = 97 ГПа, C44 = 33 ГПа, C' = = 29 ГПа, B = 117 ГПа) [44] хорошо совпадают с результатами для идеальной (C11 = 154 ГПа, C12 = = 105 ГПа, C44 = 17 ГПа, C ' = 24 ГПа, B = 121 ГПа) и релаксированной (C11 = 167 ГПа, C12 = 103 ГПа, C44 = 31 ГПа, C' = 32 ГПа, B = 125 ГПа) решеток для упругих переменных, кроме С44 для идеальной ячейки; результаты С44 для релаксированной структуры очень близки к экспериментальным.
Обращает на себя внимание тот факт, что релаксация в сплавах с концентрацией CX = 25 и 50 ат. % для сплавов с элементами начала периода в меньшей степени изменяет объемный модуль B, при этом модули Юнга E и сдвига G существенно увеличиваются, особенно для концентрации 25 ат. %. Можно сделать предположение, что средняя валентная электронная плотность в этих сплавах меняется слабо, однако происходит ее перераспределение во время релаксации с формированием ковалентной компоненты атомной связи, о чем свидетельствует значительное увеличение соотношения G/B (и уменьшение ν) для Nb и Mo (Ta и W).
Расчеты показали уменьшение параметра решетки для всех элементов, причем чем выше концентрация X, тем параметр ниже. Это согласуется с соотношением радиусов атомов, поскольку радиусы всех элементов X меньше радиуса циркония. Эффект релаксации проявляется в уменьшении параметра решетки относительно идеальной структуры. Более высокая стабильность сплавов с легирующими элементами середины и конца периодов (за исключением Zr–50Ir(Rh)) может быть частично связана с меньшим параметром решетки и, соответственно, длиной межатомной связи, поскольку меньшее расстояние обеспечивает большее перекрытие d‑состояний, участвующих в межатомной связи. Зависимостью от длины межатомной связи можно также объяснить то, что значения практически всех констант Cij, а также модулей B, E, G для 5d-легирующих элементов выше, чем для 4d-элементов, из‑за большей пространственной протяженности 5d-орбиталей.
Проведем краткое сравнение свойств титановых и циркониевых сплавов. При низкой концентрации второго элемента сплавы Zr 6.25Nb и Zr 6.25Ta имеют положительные значения C ', в то время как в титановых сплавах с этими элементами C ' < 0. Для остальных сплавов Zr–X, наблюдаются либо практически одинаковые значения C ' (X = Mo и W), либо меньшие значения C ' (X = Tc, Ru, Rh; Re, Os, Ir) по сравнению с соответствующими Ti–X сплавами. Энтальпия смешения имеет в циркониевых сплавах бóльшие положительные значения для (Nb, Mo; Ta, W) и меньшие отрицательные (Tc, Ru, Rh; Re, Os, Ir), чем в титановых сплавах. Константы C11, C12, объемные модули B и модули Юнга E в Zr–6.25X-сплавах примерно на 20–25 ГПа меньше, чем в Ti–6.25X, С44 и модуль сдвига G – на 10–15 ГПа, при этом Zr–6.25X-сплавы более анизотропны.
Для высоких концентраций (25 и 50 ат. %) расчеты показали, что все упругие постоянные и поликристаллические модули в Ti–X-сплавах выше, включая C', что означает более высокую механическую стабильность. Разница в константах C11, C12, модулей Юнга E в Zr–25(50)X-сплавах достигает 50 ГПа в сторону уменьшения относительно Ti–25(50)X, в C ',С44, объемных модулях B и модулях сдвига G – ~20 ГПа, константы анизотропии снижаются примерно одинаково. Уменьшение упругих констант и поликристаллических модулей в Zr–X сплавах относительно Ti–X сплавов может быть частично связано с увеличением параметров решетки, которые в циркониевых сплавах ~ на 5–9% больше, чем в титановых. Термодинамическая стабильность также выше в титановых сплавах, поскольку значения энтальпии смешения более отрицательные, чем для Zr–X сплавов, эффект структурной оптимизации выражен в большей степени в Zr–X-сплавах. При отмеченных выше отличиях, обнаруженных в Ti–X и Zr–X-сплавах, общие зависимости изменения свойств при увеличении концентрации X либо при движении вдоль периодов достаточно похожи между собой.
ВЫВОДЫ
Результаты, полученные методом ЕМТО‑CPA, показали, что сплавы с 3d-элементами термодинамически нестабильны во всем концентрационном интервале. Энтальпия смешения ОЦК сплавов циркония с элементами середины 5 и 6 периодов отрицательна, тогда как легирование металлами начала и конца периода демонстрирует термодинамическую неустойчивость этих сплавов.
Показано, что сплавы циркония с элементами пятого и шестого периодов имеют больший диапазон значений упругих констант С ', С11, С12, С44, и модулей упругостей E и G, чем сплавы с 3d-элементами. Максимальные значения модуля Юнга и модуля сдвига для всех концентраций наблюдаются для сплавов с элементами середины периода для 4d и 5d-металлов Tc, Ru; (Re Os), тогда как при легировании 3d-металлами максимум смещается в сторону Co, Ni. Анализ периодических зависимостей константы С' позволяет сделать вывод о том, что элементы середины периодов Fe, Co, Ni (3d), Tc, Ru, Rh (4d) и Re, Os, Ir (5d) обладают наибольшей стабилизирующей способностью. При этом 4d- и 5d-металлы являются более сильными стабилизаторами ОЦК-структуры Zr. Предполагается, что магнитное взаимодействие в сплавах циркония с 3d-металлами оказывает существенное влияние на термодинамические и упругие свойства и требует более глубокого исследования.
Расчеты методом PAW‑SQS показали, что сплавы с низким содержанием второго компонента X (6.25 ат. %) имеют очень низкие значения константы C ' и высокие значения параметра анизотропии, что указывает на неустойчивость ОЦК-твердого раствора Zr–6.25X. Значения модуля Юнга не превышают 40 ГПа, модуля сдвига 15 ГПа. При увеличении концентрации X (25 ат. %) рост упругих констант C11, C ', и как следствие, стабильности системы наблюдается для всех элементов, с максимальным эффектом для Re, Os (Tc, Ru). Для этих элементов также значительно увеличиваются константы C44, модули Юнга E и сдвига G имеют значения ~150 и ~70 ГПа соответственно. Для высоких концентраций второго компонента (50 ат. %) сплавы с элементами середины периода Re (Тс) показывают максимум стабильности ОЦК-кристалла. Одновременно со стабильностью высокие механические характеристики демонстрируют сплавы c Re, Os (Tc, Ru), для которых модули Юнга и сдвига достигают значений ~200 и ~80 ГПа соответственно.
Максимальное увеличение при легировании испытывает константа C11, это демонстрирует, что добавление второго компонента больше усиливает устойчивость к осевой деформации, чем к деформации сдвига.
Анализ параметров пластичности показывает, что сплавы обладают преимущественно металлическим типом атомной связи, при этом с увеличением концентрации Х увеличивается доля ковалентной составляющей и растет ионный вклад для элементов середины периода.
Показано, что оптимизация ОЦК-структуры увеличивает стабильность и упругие характеристики сплавов за счет перераспределения электронной плотности и увеличения направленности связи. Однако при этом важен контроль сохранения позиций ОЦК-структуры, который может не выполняться, несмотря на термодинамическую стабильность систем, как в случае Zr–50Ir и Zr–50Rh сплавов.
Сравнение с титановыми сплавами показывает, что Zr–X-сплавы имеют похожие тенденции изменения свойств при движении по периодам и при увеличении содержания второго компонента, более механически стабильны для сплавов с низким содержанием Nb, Ta и Mo, но с небольшим понижением термодинамической стабильности, значений упругих постоянных и поликристаллических модулей для всех рассматриваемых концентраций X.
При сравнении результатов, полученных методами EMTO-CPA и PAW-SQS, показано, что оба метода воспроизводят тенденции изменения упругих свойств. Параметры решетки, полученные обоими методами, практически совпадают, а значения энтальпии близки. Основными несоответствиями между методами является то, что EMTO-CPA не воспроизводит область нестабильности по C ' при нулевой и низких концентрациях второго элемента, показывает завышенные значения упругой константы С44 и менее пластическое поведение исследуемых сплавов относительно результатов PAW-SQS. Для повышения точности результатов EMTO-CPA по упругим и механическим характеристикам, эти результаты могут быть скалированы с учетом результатов PAW-SQS для чистого циркония и некоторых выбранных реперных точек. Методология такого скалирования большого набора неточных и нересурсоемких данных на малый набор точных и ресурсоемких данных представляет собой актуальную задачу, так как может сильно сократить время расчета свойств с высокой точностью.
В заключение отметим, что в работе предсказан весь возможный диапазон значений упругих и механических характеристик, которые могут быть достигнуты в ОЦК-бинарных циркониевых сплавах с переходными металлами, а также с Al, In и Sn при низких температурах. Поэтому дальнейшими направлениями исследований в данной области могут быть исследования температурных зависимостей указанных характеристик бинарных сплавов, что особенно актуально для предсказанных составов с низкой C ', т.к. в таких сплавах следует ожидать мартенситное превращение и возможный эффект памяти формы.
Работа выполнена при поддержке Российского Научного Фонда (проект № 21-72-10105 https:// rscf.ru/project/21-72-10105. Национальный исследовательский технологический университет “МИСИС”, г. Москва). Расчеты проведены на суперкомпьютерном кластере НИТУ МИСИС.
Список литературы
Banerjee S., Mukhopadhyay P. Phase Transformations: examples from titanium and zirconium alloys // Pergamon Mater. Series. 2007. 813 p.
Добромыслов А.В. Влияние D-металлов на температуру полиморфного и (моно) эвтектоидного превращения в бинарных сплавах титана, циркония и гафния // ФММ. 2020. Т. 121. № 5. С. 516–521.
Белозерова А.Р., Белозеров С.В., Шамардин В.К. К вопросу моделирования эффектов ядерной трансмутации при исследовании физических свойств циркониевых сплавов // ФММ. 2020. Т. 121. № 6. С. 564–575.
Рожнов А.Б., Рогачев С.О., Ханан Алшеих, Просвирнин Д.В. Циклическая прочность сплава Zr–1% Nb после равноканального углового пресования // ФММ. 2022. Т. 123. № 1. С. 109–116.
Егорова Л.Ю., Хлебникова Ю.В., Пилюгин В.П., Реснина П.П. Калориметрия и особенности обратного ω → α-фазового превращения в псевдомонокристаллах Zr и Ti // ФММ. 2022. Т. 123. № 5. С. 515–521.
Рогачев С.О., Андреев В.А., Горшенков М.В., Тен Д.В., Кузнецова А.С., Щербаков А.Б. Улучшение прочностных характеристик сплава Zr–2.5% Nb ротационной ковкой // ФММ. 2022. Т. 123. № 9. С. 1002–1008.
Скворцов А.И., Скворцов А.А. Влияние температуры старения на параметры амплитудной зависимости внутреннего трения твердость и структуру сплава Zr–8.1% Nb // ФММ. 2022. Т. 123. № 11. С. 1175–1181.
Фирсова А.Г, Табачкова Н.Ю., Базлов А.И. Влияние высокотемпературной прокатки и отжига на структуру и свойства аморфного сплава на основе циркония // ФММ. 2021. Т. 122. № 8. С. 845–850.
Suzuki A.K., Campo K.N., Fonseca E.B., Araújo L.C., Gandra F.C.G., Lopes E.S.N. Appraising the potential of Zr-based biomedical alloys to reduce magnetic resonance imaging artifacts // Sci. Reports. 2020. V. 10. P. 2621.
Niinomi M. Recent titanium R&D for biomedical applications in japan // JOM. 1999. V. 51. P. 32–34.
Li Y., Wong C., Xiong J., Hodgson P., Wen C. Cytotoxicity of titanium and titanium alloying elements // J. Dent. Res. 2010. V. 89. P. 493–497.
Suyalatu, Kondo R., Tsutsumi Y., Doi H., Nomura N., Hanawa T. Effects of phase constitution on magnetic susceptibility and mechanical properties of Zr-rich Zr–Mo alloys // Acta Biomater. 2011. V. 7. P. 4259–4266.
Zhou F.Y., Wang B.L., Qiu K.J., Lin W.J., Li L., Wang Y.B., Nie F.L., Zheng Y.F. Microstructure, corrosion behavior and cytotoxicity of Zr–Nb alloys for biomedical application // Mater. Sci. Eng. C. 2012. V. 32. P. 851–857.
Zhou F.Y., Wang B.L., Qiu K.J., Lin J., Li L., Li H., Zheng Y. Microstructure, mechanical property, corrosion behavior, and in vitro biocompatibility of Zr-Mo alloys // J. Biomed. Mater. Res. – Part B Appl. Biomater. 2013. V. 101. P. 237–246.
Guo S., Zhang J., Shang Y., Zhang J., Meng Q., Cheng X., Zhao X. A novel metastable β-type Zr–12Nb–4Sn alloy with low Young’s modulus and low magnetic susceptibility // J. Alloys Compd. 2018. V. 745. P. 234–239.
Guo S., Zhang J., Shang Y., Zhang J., Meng Q., Cheng X., Zhao X. A metastable β-type Zr–4Mo–4Sn alloy with low cost, low Young’s modulus and low magnetic susceptibility for biomedical applications // J. Alloys Compd. 2018. V. 754. P. 232–237.
Hinomi M. Metals for biomedical devices // Woodhead Publishing, 2019.
Kondo R., Nomura N., Suyalatu, Tsutsumi Y., Doi H., Hanawa T. Microstructure and mechanical properties of as cast Zr–Nb alloys // Acta Biomater. 2011. V. 7. P. 4278–4284.
Kondo R., Nomura N., Suyalatu, Tsutsumi Y., Doi H., Hanawa T. Effects of phase constitution on magnetic susceptibility and mechanical properties of Zr-rich Zr–Mo alloys // Acta Biomater. 2011. V. 7. P. 4259–4266.
Kobayashi E., Ando M., Tsutsumi Y., Doi H., Yoneyama T., Kobayashi M., Hanawa T. Inhibition effect of zirconium coating on calcium phosphate precipitation on titanium to avoid assimilation with a bone // Mater. Trans. 2007. V. 48. P. 301–306.
Steinbruck M. High-Temperature oxidation of zirconium alloys in various atmospheres // Encyclopedia of Materials: Metals and Alloys. 2022. P. 453–464.
Yau T.-L., Annamalai V.E. Corrosion of zirconium and its alloys // Reference module in Materials Science and Material Engineering. 2016. 39 p.
Krishnan R., Asundi M.K. Zirconium alloys in nuclear technology // Proceedings of the Indian academy of Sciences Section C: Engineering Sciences. 1981. V. 4. P. 41–56.
Kobayashi E., Matsumoto S., Doi H., Yoneyama T., Hamanaka H. Mechanical properties of the binary titanium-zirconium alloys and their potential for biomedical materials // J. Biomed. Mater. Research. 1995. V. 29. P. 943–950.
Li Y., Cui Y., Zhang F. Xu H. Shape memory behavior in Ti-Zr alloys // Scr. Mater. 2011. V. 64. P. 584–587.
Ijaz M.F, Kim H.Y, Hosoda H. Miyazaki S. Superelastic properties of biomedical (Ti–Zr)–Mo–Sn alloys // Mater. Sci. Eng. 2015. V. 48. P. 11–20.
Hickman B. S. The formation of omega phase in titanium and zirconium alloys: A review // J. Mater. Sci. 1969. V. 4. P. 554–563.
Zong H., He P., Ding X., Ackland G. J. Nucleation mechanism for hcp → bcc phase transformation in shock-compressed Zr // Phys. Rev. B. 2020. V. 101. P. 144 105.
Vitos L., Abrikosov I.A., Johansson B. Anisotropic Lattice Distortions in Random Alloys from First‑Principles Theory // Phys. Rev. Lett. 2001. V. 87. P. 156 401.
Vitos L. Computational Quantum Mechanics for Materials Engineers: The EMTO Method and Applications // Springer‑Verlag, London. 2007. 237 p.
Blöchl P.E. Projector augmented‑wave method // Phys. Rev. B. 1994. V. 50. № 24. P. 17953–17979.
Kresse G., Joubert D. From ultrasoft pseudopotentials to the projector augmented‑wave method // Phys. Rev. B. 1999. V. 59. P. 1758–1775.
Kresse G., Hafner J. Ab initio molecular dynamics for liquid metals // Phys. Rev. B. 1993. V. 47. P. 558–561.
Kresse G., Furthmüller J. Efficiency of ab‑initio total energy calculations for metals and semiconductors using a plane‑wave basis set // Comput. Mater. Sci. 1996. V. 6. P. 15–50.
Kresse G., Furthmüller J. Efficient iterative schemes for ab initio total‑energy calculations using a plane‑wave basis set // Phys. Rev. B. 1996. V. 54. P. 11169–11186.
Kollár J., Vitos L., Skriver H.L. Electronic Structure and Physical Properties of Solids: The Uses of the LMTO Method // Springer‑Verlag, Berlin. 2000. P. 85–113.
Perdew J.P., Burke K., Ernzerhof M. Generalized Gradient Approximation Made Simple // Phys. Rev. Lett. 1996. V. 77. P. 3865.
Mouhat F., Coudert F. Necessary and sufficient elastic stability conditions in various crystal systems // PRB. 2014. V. 90. P. 224104.
Zunger A., Wei S.-H., Ferreira L.G., Bernard J.E. Special quasirandom structures // Phys. Rev. Lett. 1990. V. 65. P. 353.
Skripnyak N.V., Ponomareva A.V., Belov M.P., and Abrikosov I.A. Ab initio calculations of elastic properties of alloys with mechanical instability: Application to BCC Ti–V alloys // Mater. Des. 2018. V. 140. P. 357–365.
Smirnova E.A., Ponomareva A.V., Syzdykova A.B., Belov M.P. Ab initio systematic description of thermodynamic and mechanical properties of binary bcc Ti‑based alloys // Mater. Today Comm. 2022. V. 31. P. 103 583.
Pugh S.F. XCII. Relations between the elastic moduli and the plastic properties of polycrystalline pure metals // Lond. Edinb. Dublin Philos. Mag. J. Sci. 1954. V. 45. P. 823–843.
Францевич И.Н., Воронов Ф.Ф., Бакута С.А. Упругие постоянные и модули упругости металлов и неметаллов. Киев: Наукова думка, 1982. 286 с.
Walker E., Peter M. Elastic constants of the bcc phase in niobium-zirconium alloys between 4.2 and 300 K // J. Appl. Phys. 1977. V. 48. P. 2820–2826.
Vander Voort G.F. (Ed.). ASM Handbook // Metallography and Microstructures. 2004. Chap. 9.
Wang Y., Curtarolo S., Jiang C., Arroyave R., Wang T., Ceder G., Chen L.Q., Liu Z.K. Ab initio lattice stability in comparison with CALPHAD lattice stability // Calphad. 2004. V. 28. P. 79–90.
Skripnyak N.V., Ponomareva A.V., Belov M.P., Syutkin E.A., Khvan A.V., Dinsdale A.T., Abrikosov I.A. Mixing enthalpies of alloys with dynamical instability: bcc Ti–V system // Acta Mater. 2020. V. 188. P. 145.
Skripnyak N.V., Tasnádi F., Simak S.I., Ponomareva A.V., Löfstrand J., Berastegui P., Jansson U., Abrikosov I.A. Achieving low elastic moduli of bcc Ti–V alloys in vicinity of mechanical instability // AIP Advances. 2020. V. 10. P. 105322.
Kolli R., Devaraj A. A review of metastable beta titanium alloys // Metals. 2018. V. 8. P. 506.
Körling M., Häglund J. Cohesive and electronic properties of transition metals: The generalized gradient approximation // Phys. Rev. B. 1992. V. 45. P. 13 293–13 297.
Еременко В.Р., Семенова Е.Л., Штепа Т.Д. Фазовая диаграмма системы Zr–Ir // Известия академии наук СССР: Металлы. 1980. № 5. С. 237–241.
Benites G.M., Fernandez Guillermet A., Cuello G.J., Campo Javier. Structural properties of metastable phases in Zr–Nb alloys // J. Alloys Compd. 2000. V. 299. P. 183–188.
Pearson W.B. A Handbook of Lattice Spacings and Structures of Metals and Alloys. Pergamon-Oxford, 1958. 1054 p.
Rapp Ö. Superconductivity and lattice parameters in the zirconium‑molybdenum, zirconium‑tungsten, hafnium‑molybdenum and hafnium‑tungsten alloy systems // J. Less‑Common Met. 1970. V. 21. P. 27–44.
Murray J.L. The Ti−Zr (Titanium–Zirconium) system // Bulletin of Alloy Phase Diagrams. 1981. V. 2. P. 197–201.
Bao X., Li X., Ding J., Liu X., Meng M., Zhang T. Exploring the limits of mechanical properties of Ti‑Zr binary alloys // Mater. Lett. 2022. V. 318. P. 132091.
Zhang N.‑N., Zhang Y.-J., Yang Y., Zhang P., Ge C.-C. First‑principles study of structural, mechanical, and electronic properties of W alloying with Zr // Chin. Physics B. 2019. V. 28. P. 046301.
Diyou J., Li X., Xuemei H., Tao W., Jianfeng H. Effect of Zr additions on crystal structures and mechanical properties of binary W–Zr alloys: A first‑principles study // J. Mater. Res. 2019. V. 34. P. 290–300.
Ikehata H., Nagasako N., Furuta T., Fukumoto A., Miwa K., Saito T. First‑principles calculations for development of low elastic modulus Ti alloys // Phys. Rev. B. 2004. V. 70. P. 174113.
Al‑Zoubi N., Schonecker S., Ii X., Li W., Johansson B.S., Vitos L. Elastic properties of 4d transition metal alloys: Values and trends // Comput. Mater. Sci. 2019. V. 159. P. 273–280.
Дополнительные материалы отсутствуют.
Инструменты
Физика металлов и металловедение