Микология и фитопатология, 2021, T. 55, № 2, стр. 129-137
DYNAMICS OF COMPLEXES OF MICROFUNGI IN THE PROCESS OF OVERGROWING SPOIL TIPS OF COAL MINES IN THE SOUTHERN TUNDRA ZONE (KOMI REPUBLIC)
V. A. Iliushin 1, *, I. Yu. Kirtsideli 1, **
1 Komarov Botanical Institute of the Russian Academy of Sciences
197376 St. Petersburg, Russia
* E-mail: ilva94@yandex.ru
** E-mail: microfungi@mail.ru
Поступила в редакцию 11.11.2020
После доработки 30.11.2020
Принята к публикации 23.12.2020
Аннотация
The material for this investigation was samples spoil tips of coal mines from the northern region of the Komi Republic (near the Vorkuta city). Samples were obtained from spoil tips of coal mines with different ages: formed (exploited), 10-year-old, 20-year-old and 45-year-old. 39 species of microfungi from 29 genera were isolated. An increase in the number, biomass and species diversity depending on the age of the spoil tips of coal mines were shown. Species of the genera Botrytis, Cladosporium, Penicillium, and Pseudogymnoascus were identified both in the spoil tips of coal mines and in the control soils of the southern tundra. Only in the control samples of soils from the southern tundra species from the genera Cosmospora, Glaciozyma and some other were identified. Modification of micromycete complexes was also noted, depending on the age of the spoil tips of coal mines. It is shown that microfungi complexes of the spoil tips of coal mines are much closer to each other than to the southern tundra community those. The processes of the formation of the mycobiota which is characteristic of the natural soils of this region are extremely slow.
INTRODUCTION
The northern region of the Komi Republic is in the permafrost zone. Despite the severity of the subarctic climate, this region has great economic importance (Kuznetsov et al., 2016).
Vorkuta city is located in the southern tundra zone. Despite the relative development and economic potential of this region, few studies have been devoted to the mycobiota of the ecosystems of the Polar Urals and the Urals. The soils of the northern, southern and mountainous tundra (Stenina, 1974; Khabibullina, 2009; Kirtsideli et al., 2010, 2011; Kovaleva et al., 2020) and the soils subjected to agricultural development (Khabibullina, Panyukov, 2010; Kovaleva et al., 2017) were the most investigated soils. However, the territories that were affected by the greatest anthropogenic impact, which include the territories associated with coal mining, were the least investigated territories. Thus, an investigation of the soil microbiota, plant community, and soils in the area affected by the spoil tip of the Vorkutinskaya coal mine is noted (Khabibullina et al., 2015).
Coal mining is accompanied by the destruction of natural ecosystems and the formation of new technogenic landscapes. Coal mining can spread over large areas in the case of the long-term large-scale operation of mines and open pits. The discovery of the Pechora coal basin dates to 1924, but its s development started in 1931. At the peak of economic development in the 1980s, 13 mines functioned in Vorkuta and nearby villages. This proliferation led to the transformation of significant areas of the city into various forms of landscape that consist of spoil tips of coal mines. Currently, coal is mined in a closed way by the Zapolyarnaya, Vorkutinskaya, Komsomolskaya, and Vorgashorskaya mines, as well as by open pit mining at the Yunyaginsky open pit. There is also the Pechorskaya Central Concentration Plant (Vorkuta.., 2011).
Some micromycetes are able to settle on rocky substrates in natural and technogenic ecosystems (Vlasov et al., 2005; Gadd, 2010). These technogenic ecosystems include spoil tips of coal mines. In addition to the lack of nutrients and water, microscopic fungi of spoil tips, due to the subarctic climate, are subject to prolonged exposure to low temperatures, repeated cycles of freezing and thawing, ultraviolet (UV) radiation and low humidity (Ruisi et al., 2006).
The formation of spoil tips is associated with the removal of deep mine heavy clay and sand-pebble rocks to the surface. Due to this removal, the surface layer of the spoil tip, whose heavy clay and sandy-pebble nature is the primary substrate for the growth of microfungi, is practically devoid of available organic matter. Primary succession occurs with natural overgrowth of waste dumps, and young technogenic soils are formed (Egorova et al., 2013). Soil formation begins with the weathering of the original rock, including weathering due to biogenic weathering (Frouz et al., 2011). Simultaneously, microscopic fungi have a central role in the formation of young soils and participate in biogenic weathering and the formation of humus and mycorrhiza of plants-pioneers (Detheridge et al., 2018). Note that the succession will proceed especially slowly in the Arctic, since the northern ecosystems are more vulnerable to technogenic impact and biological processes at high latitudes are decelerated (Krause-Jensen, Duarte, 2014).
Due to the presence of soil tips that formed at different times, it is possible to study the biodiversity dynamics of microfungi in spoil tips over a sufficiently long period of time. Note that reclamation was not carried out on spoil tips in the Vorkuta region, which facilitates observation of the natural succession of spoil tips.
The purpose of this study was to explore the dynamics of microscopic fungi complexes of spoil tips in coal mines during the process of their overgrowth in the southern tundra zone (Komi Republic).
MATERIALS AND METHODS
Sampling. The research materials were samples obtained at the end of July 2019 from the spoil tips of the Komsomolskaya and Zapolyarnaya coal mines and the spoil tip of the Pechora Central Processing Plant near the city of Vorkuta (67°30′ N, 64°02′ E). The samples were obtained from 10-year-old, 20-year-old and 45-year-old spoil tips of the coal mines. Samples were also taken from the spoil tip, which is still being exploited (formed). Soils of the southern tundra (dwarf-willow-cotton tundra and grass-moss tundra) were employed as the control. Geobotanical descriptions were performed on the selected sites, soil profiles were constructed, and average samples of soils and spoil tips rock were selected (Table 1).
Table 1.
Sampling locations
Samples | Mine | Horizons | Coordinates | Projective cover |
---|---|---|---|---|
Formed (exploited) spoil tip | Pechorskaya CCP | Not formed | 67°30′18″N 63°40′44″E | 0% |
10-year-old spoil tip | Zapolyarnaya | 1 (0–10 cm) | 67°29′31″N 63°46′54″E | 0% |
2 (10–40 cm) | ||||
20-year-old spoil tip | Komsomolskaya | 1 (0–10 cm) | 67°33′09″N 63°50′40″E | 1–2% |
2 (10–40 cm) | ||||
45-year-old spoil tip | Komsomolskaya | 1 (0–10 cm) | 67°35′51″N 63°50′06″E | 15% |
2 (10–20 cm) | ||||
3 (20–40 cm) | ||||
Control (soils of southern tundra) | 1 (0–3 cm) | 67°27′09.9″N 64°01′08.3″E | 100% | |
2 (3–20 cm) | ||||
3 (20–50 cm) |
Samples of rock and soil for mycological analysis were collected in individual sterile 50-milliliter plastic tubes. The depth of sampling was determined by the soil horizons. All samples were stored at 4°C.
Isolation and identification. Fungi were isolated by serial dilutions followed by sowing a soil or rock suspension on a Czapek’s agar (CZA) (Raper, Thom, 1949; Goldman, Green, 2015). The fungi were cultivated in the dark at 20°C and 4°C. Chloramphenicol (100 mg/L) was added to the culture medium to suppress the growth of bacteria. The initial identification of fungal isolates was carried out based on the morphological characteristics using the most common determinant after their isolation in a pure culture (Domsch et al., 2007). For micromorphological examination, microscopy by Carl Zeiss AxioImager А1 was employed.
Some isolates were also identified by molecular methods. The cultures employed for molecular studies were cultivated on Czapek’s agar (CZ) at 20°C for 14 days. Deoxyribonucleic acid (DNA) was extracted by using a Diamond DNA Plant kit (ABT, Russia, Barnaul) according to the manufacturer’s instructions. The internal transcribed spacer (ITS) region was applied as a phylogenetic marker. ITS rDNA region (ITS1–5.8S–ITS2) was amplified using the polymerase chain reaction (PCR) primers ITS1 (5'-TCC-GTA-GGT-GAA-CCT-TGC-GG-3') and ITS4 (5'-TCC-TCC-GCT-TAT-TGA-TAT-GC-3') (White et al., 1990). Upon completion of the amplification, the samples were detected by an electrophoretic method in 1.5% agarose gel with GelRed. The obtained DNA fragments were sequenced by the commercial company BioBeagle (St. Petersburg, Russia) via the Sanger method. Sequences were inspected and assembled using BioEdit version 7.1.9. Newly generated sequences were compared to the available sequences in the GenBank database (National Center for Biotechnology Information, NCBI) by using the Basic Local Alignment Search Tool (BLAST) instrument (http://blast.ncbi.nlm.nih.gov/Blast.cgi). In addition, the followed criteria were applied to interpret the sequences from the GenBank database: for query coverage and sequence identities ≥98%, the genus and species were accepted; for query coverage and sequence identities between 95% and 97%, only the genus was accepted (Godinho, 2013).
The names and positions of the taxa of microfungi were unified using the database www.indexfungorum.org/Names/fungic.asp.
Characterisation of the complexes of fungi. Colonies were counted after 10 days (20°C) of cultivation and 30 days (4°C) of cultivation. Data on the total number of micromycetes were expressed in colony-forming units per 1 g of absolutely dry soil (CFU/g). Biomass indicators were determined by luminescent microscopy in accordance with the generally accepted method (Hoch et al., 2005). To characterise the structures of fungal complexes, the relative abundance of species and genera were employed (Magguran, 1988, 2004).
The Shannon index of Diversity and Pielou index were utilised to characterise the structure of a fungi biota (Magguran, 1988, 2004). To study the similarity of micromycete complexes, we used the tree clustering method, where the distances between two clusters were estimated using the Ward method (Ward, 1963). To estimate the expected number of species in areas studies and the completeness of the study, we employed the approach developed by the Gotelli, Colwell and Chao (Colwell et al., 2012) based on an algorithm for generating samples.
Statistical processing was performed using the statistical software package MS Excel 2007, Statistica 10.0 and EstimateS 9.
RESULTS AND DISCUSSION
Analysis of the structures of microfungi complexes. From the research, 39 species of microscopic fungi were identified (Table 2); they belong to 29 genera. The specific abundance of the main genera of fungi in the spoil tips of the coal mines is shown in Figure 1.
Table 2.
Micromycetes isolated from spoil tips of coal mines and soils of the tundra (control) CFU/g at 20°C
Species | Accession number | Formed spoil tip | Horizons of 10-year-old spoil tip | Horizons of 20-year-old spoil tip | Horizons of 45-year-old spoil tip | Horizons of control (soils of south tundra) | ||||||
---|---|---|---|---|---|---|---|---|---|---|---|---|
1 | 2 | 1 | 2 | 1 | 2 | 3 | 1 | 2 | 3 | |||
Acideaextrema Hujslová et M. Kolařík | MW176126, MW176124 | 0 | 0 | 0 | 9 | 47 | 0 | 0 | 0 | 0 | 0 | 0 |
Alternariaconsortialis (Thüm.) J.W. Groves et S. Hughes | 0 | 0 | 0 | 0 | 0 | 0 | 0 | 0 | 35 | 0 | 0 | |
Aspergillusflavus Link | 0 | 0 | 0 | 0 | 0 | 0 | 0 | 0 | 0 | 35 | 35 | |
A.fumigatus Fresen. | 0 | 0 | 0 | 0 | 0 | 9 | 0 | 0 | 35 | 0 | 0 | |
Botrytiscinerea Pers. | 0 | 0 | 0 | 0 | 0 | 103 | 93 | 75 | 0 | 0 | 0 | |
B.elliptica (Berk.) Cooke | MW176125 | 28 | 28 | 35 | 9 | 0 | 383 | 47 | 28 | 2520 | 1050 | 700 |
Cladosporiumcladosporioides (Fresen.) G.A. de Vries | 5 | 7 | 0 | 9 | 0 | 0 | 0 | 0 | 630 | 140 | 35 | |
C.herbarum (Pers.) Link | 0 | 0 | 0 | 0 | 0 | 0 | 0 | 0 | 105 | 35 | 35 | |
C.sphaerospermum Penz. | 9 | 14 | 49 | 103 | 19 | 47 | 9 | 9 | 70 | 175 | 35 | |
Coniochaetahoffmannii (J.F.H. Beyma) Z.U. Khan, Gené et Guarro | MW176128 | 0 | 0 | 0 | 0 | 0 | 37 | 9 | 0 | 0 | 0 | 0 |
Cordycepsfarinosa (Holmsk.) Kepler, B. Shrestha et Spatafora | 0 | 0 | 0 | 65 | 9 | 0 | 0 | 0 | 0 | 0 | 0 | |
Cosmosporaberkeleyana (P. Karst.) Gräfenhan, Seifert et Schroers | 0 | 0 | 0 | 0 | 0 | 0 | 0 | 0 | 210 | 875 | 175 | |
Fusariumtricinctum (Corda) Sacc. | MW176144 | 0 | 0 | 0 | 0 | 0 | 0 | 0 | 0 | 35 | 0 | 0 |
Fusicollamerismoides (Corda) Gräfenhan, Seifert et Schroers | MW176134 | 0 | 0 | 0 | 0 | 0 | 0 | 0 | 0 | 35 | 700 | 70 |
F. ossicola Lechat et Rossman | MW176147 | 0 | 0 | 0 | 0 | 0 | 0 | 0 | 0 | 0 | 35 | 0 |
Glaciozymaantarctica (Fell, Statzell, I.L. Hunter et Phaff) M. Groenew. et Q.M. Wang | MW176139 | 0 | 0 | 0 | 0 | 0 | 0 | 0 | 0 | 2835 | 700 | 175 |
Helicodendronmultiseptatum Abdullah | MW176136 | 0 | 0 | 0 | 0 | 0 | 0 | 0 | 0 | 0 | 35 | 0 |
Juxtiphomaeupyrena (Sacc.) Valenz.-Lopez, Crous, Stchigel, Guarro et Cano | MW176135 | 0 | 0 | 0 | 0 | 0 | 0 | 9 | 0 | 35 | 0 | 0 |
Leptosphaeriasclerotioides (Preuss ex Sacc.) Gruyter, Aveskamp et Verkley | MW176131, MW176132 | 0 | 7 | 0 | 0 | 0 | 9 | 37 | 19 | 70 | 210 | 35 |
Mortierellaalpina Peyronel | MW176142, MW177782 | 0 | 7 | 42 | 0 | 28 | 19 | 9 | 9 | 0 | 0 | 0 |
Mucorhiemalis Wehmer | 0 | 21 | 28 | 0 | 0 | 0 | 0 | 0 | 315 | 140 | 70 | |
Neonectriacandida (Ehrenb.) Rossman, L. Lombard et Crous | MW176138 | 0 | 0 | 0 | 0 | 0 | 0 | 0 | 0 | 350 | 105 | 0 |
Paraboeremiaputaminum (Speg.) Qian Chen et L. Cai | 0 | 0 | 0 | 0 | 0 | 0 | 0 | 0 | 35 | 105 | 0 | |
Paraphomafimeti (Brunaud) Gruyter, Aveskamp et Verkley | MW176123, MW176148 | 0 | 0 | 0 | 9 | 9 | 0 | 0 | 0 | 735 | 210 | 70 |
Penicilliumchrysogenum Thom | MW176143 | 5 | 0 | 7 | 9 | 37 | 19 | 9 | 0 | 210 | 105 | 35 |
P.citrinum Thom | 5 | 0 | 0 | 0 | 0 | 0 | 0 | 0 | 70 | 0 | 0 | |
P.glabrum (Wehmer) Westling | 5 | 7 | 0 | 56 | 9 | 28 | 19 | 19 | 455 | 175 | 175 | |
P.janczewskii K.W. Zaleski | 0 | 0 | 0 | 0 | 0 | 187 | 37 | 28 | 700 | 350 | 175 | |
P.jensenii K.W. Zaleski | 14 | 0 | 0 | 9 | 0 | 0 | 0 | 0 | 140 | 35 | 35 | |
P.thomii Maire | MW176140 | 0 | 0 | 0 | 0 | 9 | 0 | 0 | 0 | 0 | 35 | 0 |
Phaeosphaeriaculmorum (Auersw.) Leuchtm. | MW176127 | 0 | 0 | 0 | 47 | 19 | 0 | 0 | 0 | 0 | 0 | 0 |
Phialocephalalagerbergii (Melin et Nannf.) Grünig et T.N. Sieber | MW176146 | 0 | 0 | 0 | 0 | 0 | 0 | 0 | 0 | 35 | 105 | 0 |
Phomaherbarum Westend. | MW176129, MW176130, MW176141 | 0 | 0 | 0 | 9 | 0 | 849 | 196 | 28 | 105 | 0 | 0 |
Plenodomushendersoniae (Fuckel) Gruyter, Aveskamp et Verkley | MW176133 | 0 | 28 | 7 | 0 | 0 | 0 | 0 | 0 | 0 | 0 | 0 |
Pseudogymnoascuspannorum (Link) Minnis et D.L. Lindner | MW176137, MW176149 | 5 | 49 | 77 | 56 | 121 | 187 | 93 | 56 | 5390 | 1400 | 525 |
Talaromycesfuniculosus (Thom) Samson, N. Yilmaz, Frisvad et Seifert | 0 | 0 | 0 | 0 | 0 | 0 | 0 | 0 | 175 | 70 | 35 | |
Trichocladiumcrispatum (Fuckel) X. Wei Wang et Houbraken | MW176145 | 0 | 0 | 0 | 0 | 0 | 0 | 0 | 0 | 0 | 70 | 0 |
Trichodermaaureoviride Rifai | 0 | 0 | 0 | 9 | 19 | 0 | 0 | 0 | 0 | 35 | 35 | |
T.viride Pers. | 0 | 0 | 0 | 9 | 0 | 0 | 9 | 0 | 0 | 0 | 0 |
Eight species were identified in the formed (exploited) spoil tip, which belongs to the genera Botrytis, Cladosporium, Penicillium, and Pseudogymnoascus. Isolates of the genera Botrytis and Penicillium accounted for 38% each, whereas Cladosporium accounted for – 18%. The genus Botrytis was represented by only one species – Botrytis elliptica. The percentage of Pseudogymnoascus pannorum isolates was 6%.
Representatives of 10 species from 9 genera were identified in samples of a 10-year-old spoil tip. The percentage of Pseudogymnoascus pannorum isolates was 30%. The percentage of micromycetes of the genus Cladosporium was 17%, whereas that of the genus Botrytis was 15%.
In the soil of a 20-year-old spoil tip, the species diversity of fungi increased to 16 species from 11 genera. It was also dominated by the genus Pseudogymnoascus and Cladosporium. The percentage of isolates of these species was 24% and 18%, respectively, whereas the percentage of isolates of the genus Penicillium was 18%. A remarkable fact is the discovery of the acidophilic species Acidea extrema in a 20-year-old spoil tip.
Earlier this species was detected in various extreme ecosystems, such as the coal mines of the Czech Republic and Antarctic deserts and tailings of the cinnabar deposit in the Altai Republic (Hujslova et al., 2014; Ilyushin, 2017).
The species diversity in the 45-year-old spoil tip was 14 species from 11 genera. The species of the genus Phoma (39%) dominated in this spoil tip. The percentage of Penicillium (13%), Pseudogymnoascus (12%) and Botrytis (27%) remained significant. The percentage of fungi of the genus Cladosporium has dropped significantly to 2%.
As a result of the studies that were carried out, 30 species of microscopic fungi were identified in the control samples (southern tundra soil) (Table 2), which belong to 23 genera. The species diversity in the soils of the southern tundra near Vorkuta was substantially higher than that in the rock of spoil tips. The dominant species were Pseudogymnoascus pannorum (30%), Botrytis elliptica (17%) and Glaciozyma antarctica (15%). The latter is a yeast characteristic of Antarctica (Li et al., 2020). The discovery of this species in the northern region of the Komi Republic enables it to be classified as a bipolar species (Wirtz et al., 2008). The control also included a significant percentage of microscopic fungi of the genera Cladosporium (5%), Cosmospora (5%) and Paraphoma (4%).
The presence of Pseudogymnoascus pannorum was observed in all studied rock of spoil tips and soils. Moreover, in most of the spoil tips and control soils that we studied, this species was dominant with a percentage of 12–30%. In the formed spoil tip, its percentage is only 6%, which apparently, is due to the instability of the newly formed community. This species is noted as dominant in Arctic and Antarctic soils and permafrost samples. This species is an oligotroph that can quickly populate substrates that are unsuitable for other species (Hayes, 2012).
Note the high percentage of fungi of the genus Cladosporium in young spoil tips. In the formed, 10-year and 20-year spoil tips, the percentage of fungi of this genus was at the level of 17–18%. Micromycetes of the genus Cladosporium are distributed throughout our planet and occupy many ecological niches (Bensch et al., 2012).
Number of microfungi and biomass. The number of microfungi (CFU/g) in the spoil tips of the coal mine was relatively low (Fig. 2). This indicator increased with the age of the spoil tips. Thus, in the formed (exploited) spoil tip, the quantity of microscopic fungi was on average 12 times lower than that in the 45-year-old spoil tip at 20°C. The number of microscopic fungi in the spoil tips was significantly lower than that in the control (southern tundra soil). When cultivated at 4°C, the regularities number of microfungi growth with increasing age of the spoil tips of the coal mine is preserved. However, the number of microfungi in this cultivation regime was lower since fungi did not develop, for which the minimum growth temperature was higher than 4°C.
The biomass in the spoil tips of the coal mine was also relatively low. This indicator increased with the age of the spoil tips. Thus, in a 10-year spoil tip, the biomass of microscopic fungi was on average 4 times lower than that in a 45-year spoil tip and amounted to 0.09 mg/g of rock. In the 20-year spoil tip, the biomass was already 1.4 times lower on average than that in the 45-year spoil tip and amounted to 0.26 mg/g.
The soils of the north are characterised by a predominance of propagules in the upper horizon. With depth, from the litter to the mineral horizons, the number of micromycetes decreases (Kirtsideli et al., 2011). We observed this trend in the control and the older spoil tips (45- and 20 year-old spoil tips). However, in a young 10-year-old spoil tip, the number of micromycetes increases with depth. Thus, the upper horizon contained 197 CFU/g, and then the underlying horizon contained 271 CFU/g. This finding is probably due to the formation of the spoil tip. During its formation, the rock that was on the surface and contained viable propagules was submerged under the new mine rock. Over time, the number of propagules in the upper horizon increases, which we observed for the 20-year spoil tip.
Comparison of micromycete complexes. From the data presented in the dendrogram (Fig. 3), the micromycete complexes are divided into four clusters. The first cluster combines the complexes of micromycetes that inhabit the formed spoil tip and those in different horizons of the 10-year spoil tip. The second cluster consists of micromycete complexes from a 20-year spoil tip. The third group includes micromycete complexes from different horizons of the 45-year spoil tip. The fourth cluster includes complexes of micromycetes from different soil horizons in the southern tundra (control). Moreover, the spoil tip communities are much closer to each other than to the southern tundra community. This finding indicates that the process of the formation of the mycobiota characteristic of the natural soils of this region is extremely slow.
Fig. 3.
Dendrograms of the similarity among complexes of micromycetes in spoil tips of coal mines, taking into account separation by horizons.
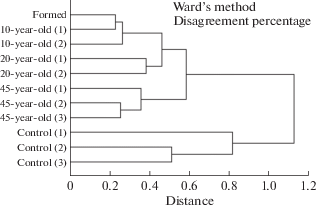
To evaluate and compare the mycobiota of spoil tips were used the Shannon index of diversity and the Pielou index (Table 3). The lowest diversity indices were observed for micromycete complexes of young spoil tips (formed and 10-year-old). With the age of the spoil tips, with the development of the succession, this indicator increases. The highest Shannon index of diversity is characteristic of the soils of the southern tundra (control). The lowest uniformity of species abundance (Pielou index) is also characteristic of the soils of the southern tundra. This is due to the simultaneous presence of pronounced dominants, such as P. pannorum, Botrytis elliptica, Glaciozyma antarctica, and a large number of rare species.
Table 3.
Shannon index of diversity (H) and Pielou evenness index (E) of mycobiota of spoil tips of coal mines (medium for horizons)
Habitats | H | E |
---|---|---|
Formed (exploited) spoil tip | 0.785224 | 0.869486 |
10-year-old spoil tip | 0.797004 | 0.797004 |
20-year-old spoil tip | 0.913081 | 0.758297 |
45-year-old spoil tip | 0.823262 | 0.718298 |
Control (soils of tundra) | 1.004004 | 0.673213 |
As indicated by the graphs of species accumulation (Fig. 4) and the values of the Chao1 index, we identified most of the expected species in the control (southern tundra soils), which comprised 91% of all expected species. However, in the spoil tips, the percentage of identified species from the expected species decreased. Moreover, the younger is the spoil tip, the lower is the detection rate probably due to the instability of the complexes and the detection of random species in them, as well as the small number of isolates in comparison with the control. Thus, for the formed (exploited) spoil tip, where the complex of micromycetes has not formed, we identified only 63% of the expected species in the graph of the species accumulation, which is not shown in the figure due to an extremely small number of isolates.
Fig. 4.
Bootstrap analysis results that show the dependence of species richness of microfungi in spoil tips and control soils from the number of investigated isolates. The thin lines show the average values of the Chao1 index (expected number of species) as the number of studied isolates increases, and the solid lines represent the smoothed curves of species accumulation, in depending on the number of isolates (along the abscissa axis, the number of isolates; along the ordinate axis, the number of species).
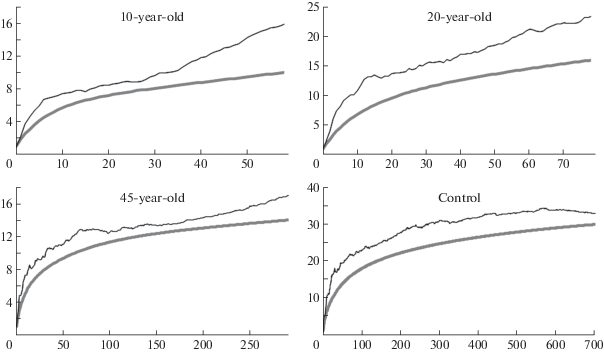
Thus, the data obtained indicate the presence of micromycetes in the spoil tips of coal mines of different ages. The differences in the complexes of micromycetes in the formed 10-year-, 20-year- and 45-year-old spoil tips are shown, taking into account their horizons. A comparison of these complexes with the complexes of the micromycetes of the southern tundra soil is also shown. The dynamics of the indicators of the number, biomass and composition of micromycete complexes with an increase in the age of the spoil tips are shown, which can be considered stages of succession. With an increase in the time elapsed after stopping the exploitation of the spoil tip, the number of species gradually increases and the number and biomass increase, which indicates an increase in the stability of the complexes and the changes that occur during the succession.
The authors are grateful to the ecologists of Vorkutaugol O.S. Geleta and N.S. Vishnyauskene for help with sampling. This study was carried out as part of the state assignment according to the thematic plan of the Botanical Institute of the Russian Academy of Sciences (theme N АААА-А19-119020890079-6). The research was done using equipment of The Core Facilities Center “Cell and Molecular Technologies in Plant Science” at the Komarov Botanical Institute RAS (St.-Petersburg, Russia).
Список литературы
Bensch K., Braun U., Groenewald J.Z. et al. The genus Cla-dosporium. Stud. Mycol. 2012. V. 72 (1). P. 1–401. https://doi.org/10.3114/sim0003
Colwell R.K., Chao A., Gotelli N.J. et al. Models and estimators linking individual-based and sample-based rarefaction, extrapolation and comparison of assemblages. J. Plant Ecol. 2012. V. 5 (1). P. 3–21.
Detheridge A.P., Comont D., Callaghan T.M. et al. Vegetation and edaphic factors influence rapid establishment of distinct fungal communities on former coal-spoil sites. Fungal Ecol. 2018. V. 33. P. 92–103. https://doi.org/10.1016/j.funeco.2018.02.002
Domsch K.H., Gams W., Anderson T.-H. Compendium of soil fungi. IHW-Verlag, Eching, 2007.
Egorova L.N., Shchapova L.N., Kovaleva G.V. et al. Soil micromycetes of technogenic landscapes in the south Primorsky territory. Mikologiya i Fitopatologiya. 2013. V. 47 (4). P. 218–222 (in Russ.).
Frouz J., Cajthaml T., Kríbek B. et al. Deep, subsurface microflora after excavation respiration and biomass and its potential role in degradation of fossil organic matter. Folia Microbiol. 2011. V. 56. P. 389–396. https://doi.org/10.1007/s12223-011-0062-9
Gadd G.M. Metals, minerals and microbes: Geomicrobiology and bioremediation. Microbiology. 2010. V. 156. P. 609–643. https://doi.org/10.1099/mic.0.037143-0
Godinho V.M., Furbino L.E., Santiago I.F. et al. Diversity and bioprospecting of fungal communities associated with endemic and cold-adapted macroalgae in Antarctica. The ISME J. 2013. V. 7. P. 1434–1451.
Goldman E., Lorrence H.G. Practical handbook of microbiology, 3rd ed. CRC Press, Boca Raton, USA, 2001.
Hayes M.A. The Geomyces fungi: Ecology and distribution. BioScience. 2012. V. 62. P. 819–823. https://doi.org/10.1525/bio.2012.62.9.7
Hoch H.C., Galvani C.D., Szarowski D.H. et al. Two new fluorescent dyes applicable for visualization of fungal cell walls. Mycologia. 2005. V. 97 (3). P. 580–588.
Hujslová M., Kubátová A., Kostovčík M. et al. Three new genera of fungi from extremely acidic soils. Mycol. Progress. 2014. V. 13 (3). P. 819–831. https://doi.org/10.1007/s11557-014-0965-3
Ilyushin V.A. Study of micromycetes of technogenic ecosystems associated with the extraction of metal ores in the Altai Republic. In: Ekologiya Rossii i sopredelnyh territoriy: Materialy XXII Mezhdunar. ekol. studencheskoy konf. Novosibirsk, 2017, p. 63 (in Russ.).
Khabibullina F.M. Soil mycobiota of natural and anthropogenically disturbed ecosystems in the northeast of the European part of Russia fungi. Dr. Sci. Thesis. Syktyvkar, 2009 (in Russ.).
Khabibullina F.M., Kuznetsova E.G., Panyukov A.N. Transformation of vegetation, soils, and soil microbiota in the impact zone of the coal mine “Vorkutinskaya”. Theoretical and Applied Ecology. 2015. 4. P. 30–37 (in Russ.). https://doi.org/10.25750/1995-4301-2015-4-030-037
Khabibullina F.M., Panyukov A.N. Microbiota transformation under the influence of agriculture in tundra zone. Theoretical and Applied Ecology. 2010. V. 3. P. 52–58 (in Russ.).
Kirtsideli I.Yu., Novozhilov Yu.K., Bogomolova E.V. et al. Microfungi complexes in soils of tundra coenoses developed on acid rocks of polar Ural. Mikologiya i Fitopatologiya 2010. V. 44 (1). P. 37–46 (in Russ.).
Kirtsideli I.Yu., Novozhilov Yu.K., Bogomolova E.V. Microfungi complexes in soils developed on basic and ultramafic rocks of polar Ural. Mikologiya i Fitopatologiya. 2011. V. 45(6). P. 513–521 (in Russ.).
Kovaleva V.A., Deneva S.V., Panyukov A.N. et al. Soil fungi as components of postagrogenic biogeocoenoses in tundra. Vestnik IB Komi NC UrO RAN. 2017. V. 3. P. 7–14 (in Russ.).
Kovaleva V.A., Deneva S.V., Lapteva E.M. Microbiological characteristics of virgin and post-agrogenic tundra soils (example of the arctic zone of the Komi Republic). Trudy Karelskogo nauchnogo tsentra Rossiyskoy Akademii Nauk. 2020. V. 5. P. 5–16 (in Russ.).
Krause-Jensen D., Duarte C.M. Expansion of vegetated coastal ecosystems in the future Arctic. Frontiers in Marine Science. 2014. https://doi.org/10.3389/fmars.2014.00077
Kuznetsov S.K., Timonina N.N., Kuznetsov D.S. Resource and value potential of mineral resources of Arctic zone of Timan-Nothern Ural region. Vestnik IG Komi NC UrO RAN. 2016. 11. P. 31–39 (in Russ.).
Li A.-H., Yuan F.-X., Groenewald M. et al. Diversity and phylogeny of basidiomycetous yeasts from plant leaves and soil: Proposal of two new orders, three new families, eight new genera and one hundred and seven new species. Stud. Mycol. 2020. V. 96. P. 17–140. https://doi.org/10.1016/j.simyco.2020.01.002
Magguran A.E. Ecological diversity and its measurement. Croom Helm. London, 1988.
Magurran A.E. Measuring biological diversity. Blackwell Publishing. Oxford, 2004.
Raper K.B., Thom C. A manual of the Penicillia. The Williams and Wilkins Company, Baltimore, 1949.
Ruisi S., Barreca D., Selbmann L. et al. Fungi in Antarctica. Rev. Environ. Sci. Biotechnol. 2007. V. 6. P. 127–141.
Stenina T.A. Biological activity of some soils of the Komi ASSR. In: Materialy po pochvam Komi ASSR, Syktyvkar, 1974, pp. 35–42 (in Russ.).
Vlasov D.Y., Abakumov E.V., Nadporozhskaya M.A. et al. Lithosols of King George Island, western Antarctica. Eurasian Soil Science. 2005. V. 38 (7). P. 681–687.
Vorkuta – a city on coal, a city in the Arctic. Respublikanskiy ekologicheskiy tsentr po izucheniyu i okhrane vostochno-evropeyskikh tundr. Syktyvkar, Komi respublikanskaya tipografiya. 2011. (in Russ.).
Ward J. Hierarchical grouping to optimize an objective function. J. Amer. Statistical Association. 1963. V. 58. P. 236–244.
White T.J., Bruns T., Lee S. et al. Amplification and direct sequencing of fungal ribosomal RNA genes for phylogenetics. In: Innis M. (eds), PCR Protocols: A guide to methods and applications. Academic Press, San Diego, Calif, 1990. P. 315–322.
Wirtz N., Printzen C., Lumbsch H.T. The delimitation of Antarctic and bipolar species of neuropogonoid Usnea (Ascomycota, Lecanorales): a cohesion approach of species recognition for the Usnea perpusilla complex. Mycol. Res. 2008. V. 112. P. 472–484. https://doi.org/10.1016/j.mycres.2007.05.006
Воркута – город на угле, город в Арктике (Vorkuta). Республиканский экологический центр по изучению и охране восточно-европейских тундр; (отв. ред.-сост. М.В. Гецен). Сыктывкар, Коми республиканская типография. 2011. 511 с.
Егорова Л.Н., Щапова Л.Н., Ковалева Г.В. и др. (Egorova et al.) Почвенные микромицеты техногенных ландшафтов на юге Приморского края // Микология и фитопатология. 2013. Т. 47. № 4. С. 218–222.
Ильюшин В.А. (Ilyushin) Изучение микромицетов техногенных экосистем, связанных с добычей металлических руд республики Алтай. Экология России и сопредельных территорий: Материалы XXII Междунар. экол. студенческой конф. Новосиб. гос. ун-т. Новосибирск: ИПЦ НГУ, 2017. С. 63.
Кирцидели И.Ю., Новожилов Ю.К., Богомолова Е.В. и др. (Kirtsideli et al.) Комплексы микромицетов в почвах тундровых ценозов, сформированных на кислых горных породах Полярного Урала // Микология и фитопатология. 2010. Т. 44. № 1. С. 37–46.
Кирцидели И.Ю., Новожилов Ю.К., Богомолова Е.В. (Kirtsideli et al.) Комплексы микроскопических грибов в почвах, сформированных на основных и ультраосновных горных породах Полярного Урала // Микология и фитопатология. 2011. Т. 45. № 6. С. 513–521.
Ковалева В.А., Денева С.В., Лаптева Е.М. (Kovaleva et al.) Микробиологическая характеристика целинных и постагрогенных тундровых почв (на примере арктической зоны республики Коми) // Труды Карельского научного центра РАН. № 5. 2020. С. 5–16.
Ковалева В.А., Денева С.В., Панюков А.Н. и др. (Kovaleva et al.) Почвенные грибы как компоненты постагрогенных биоценозов в тундре. Вестник ИБ Коми НЦ УрО РАН. 2017. № 3. С. 7–14.
Кузнецов С.К., Тимонина Н.Н., Кузнецов Д.С. (Kuznetsov et al.) Ресурсный и стоимостной потенциал полезных ископаемых арктической зоны Тимано-Североуральского региона // Вестник Института геологии Коми НЦ УрО РАН. 2016. № 11. С. 31–39.
Стенина Т.А. (Stenina et al.) Биологическая активность некоторых почв Коми АССР. Материалы по почвам Коми АССР. Сыктывкар, 1974. С. 35–42.
Хабибуллина Ф.М. (Khabibullina) Почвенная микобиота естественных и антропогенно нарушенных экосистем северо-востока европейской части России: дисс. … докт. биол. наук. Сыктывкар, 2009. 364 с.
Хабибуллина Ф.М., Панюков А.Н. (Khabibullina, Panyukov) Трансформация микробиоты под влиянием сельскохозяйственного освоения почв в тундровой зоне // Теоретическая и прикладная экология. 2010. № 3. С. 52–58.
Хабибуллина Ф.М., Кузнецова Е.Г., Панюков А.Н. (Khabibullina et al.) Трансформация растительности, почв и почвенной микробиоты в зоне воздействия породных отвалов угольной шахты “Воркутинская” // Теоретическая и прикладная экология. 2015. № 4. С. 30–37.
Дополнительные материалы отсутствуют.
Инструменты
Микология и фитопатология