Зоологический журнал, 2020, T. 99, № 12, стр. 1345-1362
Presence of Acantholeberis Lilljeborg (Cladocera, Anomopoda) in South America, with Remarks on the Taxonomy and Geographic Distribution of the Genus
J. C. Paggi a, *, Y. Herrera-Martinez b, **
a Instituto Nacional de Limnología, Consejo Nacional de Investigaciones Científicas
y Técnicas – Universidad Nacional del Litoral, Ciudad Universitaria
3000 Santa, Fe, Argentina
b Grupo de Investigación Xiuâ, Escuela de Ciencias Biológicas,Universidad Pedagógica
y Tecnológica de Colombia – UPTC
150003 Tunja, Colombia
* E-mail: juanpaggi@gmail.com
** E-mail: yimyherrera@gmail.com
Поступила в редакцию 1.03.2020
После доработки 16.03.2020
Принята к публикации 17.03.2020
Аннотация
A new species of Acantholeberis Lilljeborg1853 (Acantholeberidae) is described based on specimens from high mountain lakes in the tropical Andes of Colombia. Acantholeberis smirnovi sp. n. differs from A. curvirostris (O.F. Müller) in several features of the shape of the shell, and in the armature of limbs II, III and VI. The unexpected heterogeneity found in most of the existing more complete descriptions of the latter species regarding a number of taxonomic traits, reveals the possibility that this genus, hitherto considered as monotypic, could really be a group of several species or subspecies, this suggesting its review. The geographical distribution of this genus is analyzed and discussed, suggesting that its disjunctions could be explained through vicariance during the Mesozoic and early Neogene periods, related to the palaeocontinent Euramerica and also to more recent long-distance jump events mediated by migratory birds.
The present-day systematics of Anomopoda, mainly Radopoda, includes a number of monotypic genera and even families whose existence could be related to the relictual nature (Korovchinsky, 2006) of the group or also to insufficient knowledge about its taxonomy and geographical distribution. One of these cases could be that of Acantholeberis Lilljeborg 1853. After several taxonomic changes in the past centuries, this genus was assigned to the family Macrothricidae Norman & Brady 1887. More recently, Smirnov (1976) proposed to divide this family into four subfamilies (Ilyocryptinae, Ophyoxinae, Acantholeberinae and Macrothricinae). Currently these subfamilies were elevated to the level of families (Dumont, Silva-Briano, 1998; Kotov, 2013) so that Acantholeberis remains as the only genus of the family Acantholeberidae Smirnov 1976 sensu Dumont et Silva-Briano 1998, which is, in turn, a monotypic genus because it has only one species considered to be valid and consequently type species of the genus, A. curvirostris (O.F. Müller 1776) (Smirnov, 1976, 1992; Kotov et al., 2013). Two other species have been mentioned: A. lithuanica Dybowski et Grochowski 1895 and A. rigidus (Schödler 1846) (originally attributed to the genus Acanthocercus) but they should be regarded as a nomen nudum in the first case and a junior synonym in the second case (Smirnov, 1976, 1992).
Excluding a few records from South Asia, whose validity will be discussed later, practically all the records of this genus were located in the middle-northern part of the northern hemisphere showing a typical Holarctic pattern, in inland water bodies with acidic water.
The presence of A. curvirostris was reported from a water body in the Venezuelan tepui Marahuaka (Smirnov et al., 1995), however it has not been taken into account in the published faunistic information (Zoppi De Roa, López, 2008; Kotov et al., 2013). Probably, it was overlooked. The authors, in a paper focused on the description of a new species, Streblocerus superserricaudatus, simply listed the species, without any comments, in a table of accompanying species. We report here the presence of this genus in high altitude lakes of the eastern range of the Colombian Andes.
The aims of this research are primarily to confirm the presence of Acantholeberis in South America, to describe the specimens of this genus from the Colombian Andes as a new species, to explore the possibility that A. curvirostris is, in fact, a group of species or subspecies hitherto not recognized as such, and to examine the probable origin of the current geographical distribution of the genus.
MATERIALS AND METHODS
Site description. Studied material was collected from lakes in a Colombian tropical Andes zone between 3100 and 4030 m above sea level., at 5°10′ to 6°0.6′ of north latitude. These lakes are located in the superparamo, paramo and transition zones towards the Andean forest. The highest lakes are surrounded by azonal vegetation with “frailejones”, Espeletia spp. (Asteraceae, Espeletiinae) and the rest of the country dominated by rocky soils with high slopes, such as in Lake Pozo Negro. The landscape of the paramo areas is dominated by grasslands and extensive areas of “frailejones” that grow in the most depressed areas, with accumulation of water in the soils. The transition zones between the Andean vegetation and the paramo are dominated by shrubs and dwarf trees. Lakes located in transition areas, have rooted aquatic vegetation at the littoral zone, and tend to have a higher concentration of chlorophyll than the typical paramo oligotrophic lakes. Most of the specimens were found in ultra-oligotrophic lakes (Chlorophyll <3.0 ppb) with relatively cold waters (8.7 to 13.5°C daytime average), with low conductivity, between 3.8 and 33 µS/cm, mostly acid, with pH 4.9 to 7.1. The concentrations of dissolved inorganic nitrogen and dissolved inorganic phosphorus are low, less than 200 and 50 ppb, respectively.
The studied cladoceran was recorded from eight lakes. It was rather scarce, with abundances always lower than one individual per liter. Probably the real abundance was underestimated because the samples were taken using methods for planktonic organisms, whereas Acantholeberis is a benthic inhabitant. Apparently, the wind-generated turbulence, mainly in shallow zones of the lake, allows the re-suspension of organisms and detritus deposited on the bottom. An important part of our observations were carried out on exuviae and remains collected by the plankton net.
The above-mentioned lakes are as follows: Gloria (5°22′53″ N, 73°15′00″ W, 3125 masl), Pensilvania (5°23′01″ N, 73°14′54″ W, 3127 masl), Pozo Negro (6°05′49″ N, 72°55′08″ W, 4030 masl), Jarilla (5°10′59″ N, 73°16′34″ W, 3290 masl), San Nicolás (5°10′44″ N, 3°16′19″ W, 3310 masl), Verde (5°10′44″ N, 73°16′19″ W, 3325 masl), Cristalina (5°57′33″ N, 73°05′05″ W, 3745 masl), Media (5°57′44″ N, 73°05′08″ W, 3725 masl) (Fig. 1).
Methods. Sampling was carried out with a hand net, 30 cm diameter with mesh size 75 μm. Samples were fixed in the field with 4–10% formaldehyde. Under a binocular stereomicroscope (Motic SMZ 140S), the specimens were picked and sorted from the samples using an Irwin loop and placed on a slide with a drop of 1 : 4–1 : 5 glycerol-water mixture. Specimens remained immersed overnight in this mixture and were transferred to more concentrated solutions of glycerol in order to finally place them in pure glycerol. Dissections were carried out using fine entomological needles, additionally sharpened on a fine grit sharpening stone. Detailed observations and drawings were made using a Nikon Optiphot microscope equipped with a camera lucida.
RESULTS
Taxonomy
Order Anomopoda Sars 1865
Suborder Radopoda Dumont et Silva-Briano 1998 emend. Kotov 2013
Family Acantholeberidae Smirnov 1976 sensu Dumont et Silva-Briano 1998
Genus Acantholeberis Lilljeborg 1853
Acantholeberis smirnovi Paggi et Herrera-Martinez sp. n. (Fig. 2–5)
Fig. 2.
Acantholeberis smirnovi sp. n. from Laguna Cristalina, Colombia. Parthenogenetic female: a – adult, lateral view; b – juvenile, lateral view; c – dorsal carapace, cervical sinus and ecdysis line; d – seta of armature of anterior margin of valve, “group 1”; e – armature of ventral margin, “group 2”; f – armature of ventral margin of valve close to posteroventral angle, “group 3”; g – armature of posteroventral angle, “group 4”; h – posterior margin; I – ventral portion of head of adult specimen, with antennule and labrum; j – labral protuberance in juveniles specimens. For definition of groups of setae, size and number, see description. Lengths of scale bar for each figure, in micrometer, as follows: a – 800, b – 445, c – 150, d–h – 65, i–j – 500. FMS – fine seta, OMS – outwardly directed seta; VMS – ventrally directed setae.
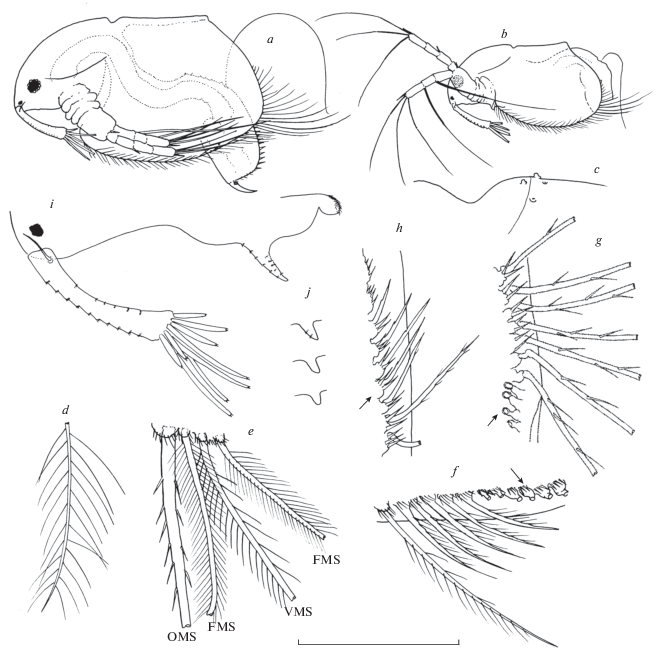
Fig. 3.
Acantholeberis smirnovi sp. n. from Laguna Cristalina, Colombia: a –postabdomen, lateral view; b – postabdomen, lateral armature, c – postabdomen armature of proximal portion, dorsal view; d – postabdominal seta; e – postabdominal claw, lateral outer view; f – lateral inner view; g– ventral view; h – antenna, setae omitted; I – lateral seta of basal segment of endopod “type I”, proximal part; j – distal part; k – apical part; l – long apical seta “type II”; m – short apical seta “type III”. Lengths of scale bar for each figure, in micrometer, as follows: a – 250, b – 120, c – 50, d – 140, e–f – 95, h – 210, i–m – 55.
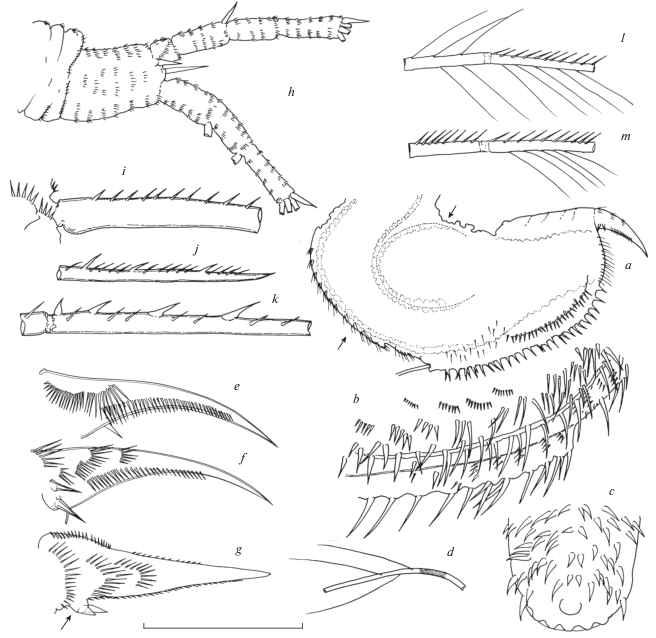
Fig. 4.
Acantholeberis smirnovi sp. n. from Laguna Cristalina, Colombia: a – right mandible; b – left mandible; c – maxilla; d – denticulate protuberance of maxilla; e – limb I, lateral outer view; f – outer distal lobe; g – inner distal lobe; h – ejector hooks; I – limb II, lateral view; j – scraper 3; k – scraper 4; l – scraper 7; m – scraper 8; n – gnathobase. Lengths of scale bar for each figure, in micrometer, as follows: a–b – 360, c – 85, d – 35, e–h – 400, f–g – 120, i – 65, j–m – 35, n – 80.
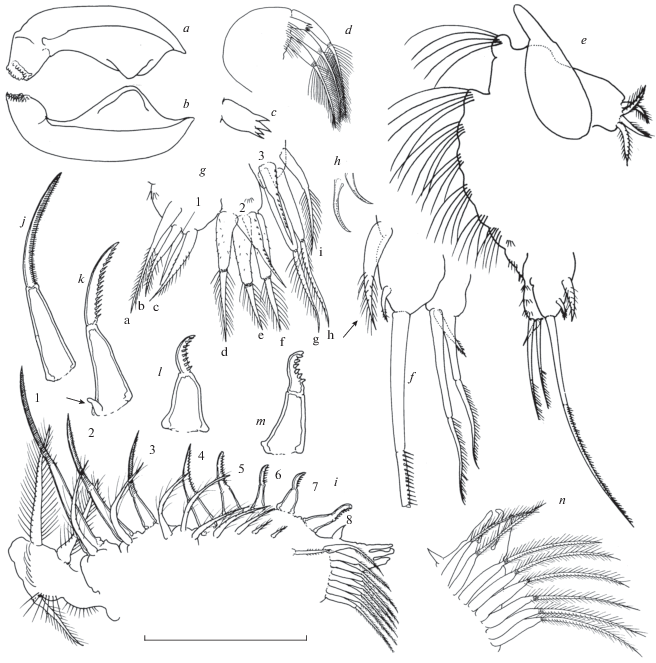
Fig. 5.
Acantholeberis smirnovi sp. n. from Laguna Cristalina, Colombia: a – limb III, exopodite; b – limb III, inner portion; c – limb IV, exopodite; d – limb IV, inner portion; e – limb V, exopodite; f – limb V, inner portion; g – limb VI. Lengths of scale bar for each figure, in micrometer, as follows: a – 240; b – 55; c, e – 205; d – 85; f – 75; g – 220.
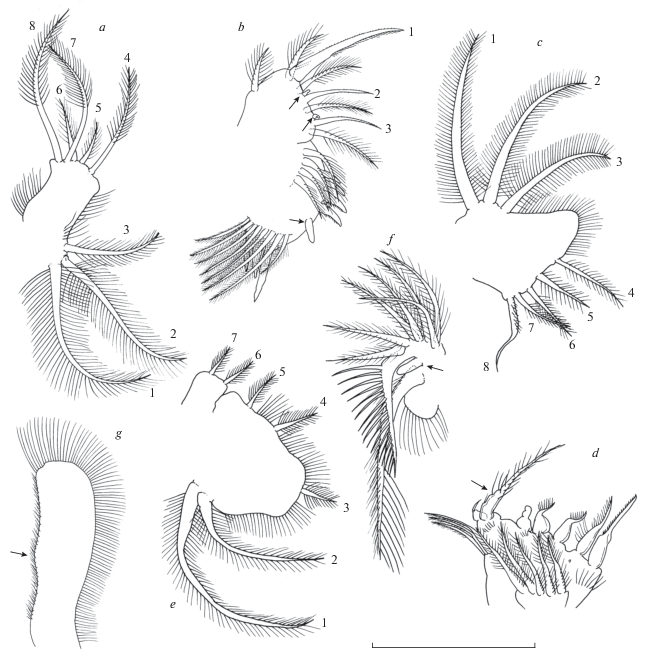
Zoobank registration number. urn:lsid:zoobank.org:pub:7F5AEC2C-7443-4936-B48C-D159DCA2B97C.
Type locality. Lake Cristalina, Paramo La Rusia, Boyacá, Colombia (5°57′33″ N 73°05′05″ W, 3745 masl).
Type material.
Holotype. Adult parthenogenetic female preserved in 4% formaldehyde, collected by Y. Herrera Martinez, deposited in the collection of the Instituto Nacional de Limnología, Santa Fe, INL20-18-1.
Paratypes. 5 undissected parthenogenetic females preserved in 4% formaldehyde, from Lake Cristalina Paramo La Rusia, Boyacá, Colombia (5°57′33″ N 73°05′05″ W, 3745 masl), collected by Y. Herrera Martinez. Deposited in the collection of the Instituto Nacional de Limnología, Santa Fe, INL20-18-2.
Etymology. This species is dedicated to Prof. Nikolai N. Smirnov, in memory of his long career, the outstanding contribution to the study of Cladocera and his generosity in sharing his valuable knowledge.
Description of parthenogenetic female. Habitus (Fig. 2a–2b). Body subrectangular-oblong in lateral view and oblong in dorsal view, 1.6–1.7 times as long as high, maximum high at middle of body length. Dorsal side practically straight in adults and somewhat concave in juveniles. Posterodorsal corner well defined, often expanded as a short blunt protuberance. Dorsal keel absent (Fig. 2a–2b).
Head (Fig. 2a–2d). Relatively large, as long as almost a half of the body length (body length/head length 0.42–0.5), subtriangular in lateral view, with dorsal side smoothly convex curved and ventral side rather straight with some blunt and short undulations and moderately concave curved and smooth in young specimens (Fig. 2a–2b). Dorsal part laterally expanded into well-developed fornices above antenna insertion and a conspicuous cervical sinus near the ecdysis line of the head (Fig. 2d). Tip of rostrum straight in frontal view. Eye relatively large, diameter about 0.25 of antennule length, distance from tip of rostrum about 1.5 diameter of eye. Ocellus diameter 0.2–0.25 diameter of eye, located close to tip of rostrum.
Labrum (Fig. 2i–2j). Relatively large, laterally compressed, with setulated apex and one conspicuous horn-like outgrowth at anterior side whose size and shape is ontogenetically variable, short and blunt in young specimens (Fig. 1 j) but long and acute in adults. In largest specimens outgrowth is armed with several transversal rows of tiny spinules.
Valve (Fig. 2a–2h). Subtrapezoidal in shape, with dorsal side shorter than ventral one (Fig. 2a–2b). Anterior, ventral and posterior margins evenly convex, posteroventral corner undefined, posterior margin connected without continuity solution with ventral margin. Posterior margin somewhat concave to straight in young specimens. Cuticle transparent, thin and delicate. Surface smooth with no distinguishable ornamentation. Occasionally a very faint reticulation is apparent, but which is no longer perceived when specimens are placed on a slide (cell contours?). Besides, in some specimens, on the dorsal surface of head and carapace some tiny verruca-like prominences or shallow punctures, scattered with an irregular arrangement, were observed (Fig. 2c). Perhaps they could be interpreted as the surficial marks produced by a parasitic or epibiont organism, e.g. the point of insertion of stalk of ciliate protozoans. Epistylis zschokkei Keiser, a peritrich protozoan has been found in Acantholeberis curvirostris (Nenniger, 1948; Bendt, 1988; Chatterjee et al., 2013). Most of the free margin of the valves, excepting the dorsalward portion of the posterior margin, covered with a continuous marginal-submarginal row of setae (about 120–125 setae) which can be classified into at least five groups, arranged from anterior to posterior sides of valve (Fig. 2d–2h). Each group is composed of a number of diversified setae which can differ between them in shape, size or both. Between one group and another group of setae there is a transitional subgroup. Herein, for describing the groups of setae, we are following the terminology and abbreviations proposed by Fryer (1974). Relative seta length is calculated as the ratio of seta length to the maximum longitudinal length of valve. Group 1: (Fig. 2d) anterior margin, 9–11 delicate setae with long and sparse setules, as long as 0.15–0.2 length of valve. Group 2: (Fig. 2d) ventral margin, 45–50 setae belonging to three different types: OMS “outwardly directed seta”, long and thick well chitinized seta with scarce mostly unilaterally short spines, FMS “fine seta”, bilaterally and densely setulated, setules long and thin and VMS “ventrally directed setae”, thicker than previous setae, with somewhat bulbose base bilaterally setulated, setules shorter and more sparse than in previous setae. There are 12–15 subgroups composed by four or three setae with the arrangement OMS-FMS-VMS or OMS-FMS-VMS-FMS. At the insertion points of each seta there are one or two spinose protuberances. Length of setae, about 0.25–0.40 length of valve. Group 3: (Fig. 2f) distalmost portion of ventral margin, 5–7 setae with unilateral row of spinules, shorter than previous row, about 0.10–0.12 length of valve. Group 4: (Fig. 2g) posteroventral “angle”, 28–32 setae somewhat stiffer and stronger than preceding setae armed with relatively spaced spines, they increase in size in a posterior direction up to middle of row decreasing then in same direction, longest setae reach about 0.55–0.6 length of valve. These setae are connected to carapace by mean of a sort of caliciform peduncle which remains attached to carapace when setae are separated out (Fig. 1f, 2g, arrows). According to Fryer (1974), in live specimens, these setae are widely splayed, outwardly directed at different angles, presumably as a defense against small predators. Taking into account the shape and thin thickness of the cuticle of these peduncles, it is plausible to assume that they function as a hinge that allows lateral movement of setae. Connection between peduncles and setae seems not to be very firm because in majority of studied specimens several setae of this group were missing. So, about it should be taken into account that our illustrations of general shape were “restored” with observations from other specimens. Group 5 (Fig. 1h): At the final part of the preceding row, pedunculated bases become reduced and turned into rounded protuberances with relatively large spines (Fig. 1h, arrow) followed by 6–7 short and robust setae which at end of row disappear remaining only spiny protuberances.
Intestine without convolutions but with a conspicuous roundish diverticulum laying in distal ventral part of intestine at beginning of postabdomen. Anus located at posterior end of postabdomen (Fig. 3a, arrow).
Postabdomen (Fig. 3a–3g). Massive, almost as wide as high, and relatively long, its length, measured from base of postabdominal setae to base of claws, as long as 0.31–0.36 of body length. Suboval in lateral view with ventral margin straight and dorsal margin as well as posterior side evenly curved. In posterior view it looks trilobate with central lobe more pronounced than lateral ones (Fig. 3a). A lateral view shows that trilobate morphology is limited to distal half of the postabdomen. Dorsal side and dorsalmost lateral side densely armed with strong spines of, at least two types, biggest ones alone or in groups of 2 or 3 members, and shorter ones forming rows of about 5 to 10 members (Fig. 3b). Spines surrounding anus are somewhat more delicate and those at proximal end are particularly robust, longest ones are located at central lobe (Fig. 3c). Dorsal part of abdomen that precedes the postabdomen is also densely armed with spines of similar size (Fig. 3a, arrow). Postabdominal seta is rather long, about 2.5 times longer than postabdomen, with some few long and thin setules (Fig. 3a, 3d). Postabdominal claws robust and relatively short, about 0.30 as long as postabdomen, with two basal spines at outer side, they both similar in length and about 0.15 as long as claw. In a ventral view they seem to arise from a low protuberance on the outer flank (Fig. 3g, arrow). Outer lateral side of claw (Fig. 3f) with 3–5 transversal rows of setules and, at dorsalmost part, one long row of setules extended along a half of claw length. Inner side of claw (Fig. 2f) with two fan-like rows of long setules at base and one long longitudinal row of setules higher in extension and size than that of outer side (Fig. 3e).
Antennule (Fig. 2i) Rod-like, slightly curved, widened distally, as long as 0.25–0.29 of body length. Sensory seta at a distance from the proximal tip of about 0.17–0.18 length of antennule. Anterior face with 8–12 transversal rows of spinules and posterior side with 6–7 similar rows of spinules. Nine relatively long aesthetascs, bifid at tip, unequal in length, 4 long and 5 short, longest ones about 0.6 length of antennule corm.
Antenna (Fig. 3h–3m). Coxal part massive and bent at right angle, integument transversally folded with row of setules at ridges and two short sensory setae at dorsolateral side; basal part robust, densely covered with transversal rows of setules, with short inner spine and a longer at outer side. Branches similar in length, all segments covered many transversal rows of spinules, basal segment of endopod robust, wider than the rest of antennal segments. Swimming setae 0–0–0–3/1–1–3, spines 0–1–0–1/0–0–1. Apical swimming setae in both branches, are two long (as long as 1.6–1.7 length of branch) and one somewhat shorter. Distal lateral swimming seta of endopod somewhat longer than longest apical seta and proximal lateral seta stronger and longer than rest of swimming setae, 2.5 as long as endopod branch. All swimming setae biarticulate with some differences and similarities in armature both in proximal as in distal segment according three types of patterns, identified as I, II and III, in brackets the corresponding seta. Type I (Fig. 3i–3k) long soft setules absent, basal and distal segments with a continuous row of short stiff setules on one side, opposite side naked in both segments (Fig. 3i–3k), distal segment with a conspicuous almost parallel row of 14–16 sparse strong spines gradually increasing in size to middle part of segment and then decreasing size towards final tip (Fig. 3i–3k). Parallelism of both rows progressively decreases distad so that at final part of short stiff setulae and strong spines are practically interspersed (Fig. 3k). Arrangement of members of rows follows an opposite trend to that of size, one or two setules between two spines at the beginning and four-five at the end of rows. Ratio distance between bases of spines/diameter of seta: mean = 3.6, maximum = 5.8, minimum = 1.4, n = 72 (lateral seta of basal segment of endopod). Type II (Fig. 3l) basal segment with bilateral row of long soft setules, distal segment with long soft setules on one side and short stiff setules on opposite side (long apical setae of both branches and distal lateral of endopod). Type III (Fig. 3m) basal and distal segments with a continuous row of short stiff setules on only one side, distal segment with row of long soft setules on opposite side (shorter apical seta in both branches).
Mandible (Fig. 4a–4b). Boat-shaped with anterior margin evenly curved and a well developed apodeme at posterior part. Masticatory surface with about twenty sclerotized diagonal ridges.
Maxilla I (Fig. 4c–4d). Corm semicircular, with three biarticulate and bilaterally setulated setae somewhat longer than corm, and one short stout protuberance with fourth apical teeth (Fig. 4d). Continuous row of setules at bases of protuberance and setae.
Limb I (Fig. 4–4h). Large, with seven transverse rows of very long setules on ventral side (Fig. 4e) and two ejector hooks armed with row of short robust denticles at inner side (Fig. 4h). Epipodite small, suboval in shape. ODL (Fig. 4f) conical with two setae, a long biarticulate seta, as long as 1.5 the length of corm, with distal segment unilaterally setulated with short setules and a very short seta armed with tiny setules at distal part. At base of outer distal lobe there is an accessory seta somewhat longer than lobe and densely setulated (Fig. 4f, arrow). Inner distal lobe (Fig. 4g) with three setae, one unsegmented seta about as long as outer distal lobe with a row of short spinules and two setae biarticulate with distal segment unilaterally setulated with short setules, as long as three and four times length of shorter one (Fig. 4g). Endite 4 with three unsegmented soft posterior setae, seta “a” 1.5 longer than “b” and “c”, all three setae unsegmented bilaterally setulated by short setules only at distal part in “a” and “b”; stiff anterior seta 1 narrower and somewhat shorter than “c” with tiny spinules at distal part. Endite 3 with three robust biarticulate soft posterior setae similar in length with distal segment bilaterally setulated and proximal segment covered with sparse tiny spinules; stiff anterior seta 2 robust, bottle-shaped, wide at base with a row of short spinules along all the length of seta. Endite 2 with four soft posterior setae, all them biarticulate with distal part bilaterally setulate with fine setules, setae “g” and “h” about 1.5 longer than “i” and “j” all them narrower than those of endite 3; stiff anterior seta 3 similar to that of endite 3 but not so robust. Endite 1 subconical somewhat elongate with four short setulated soft setae with unequal pattern of setulation (Fig. 4e).
Limb II (Fig. 4i–4n). Epipodite subconical elongated about twice longer than exopod. Exopodite suboval, with distal end expanded into a round protuberance, with some rows of setules and three soft setae, two of them bilaterally setulated with long fine setules at posterior side, proximalmost seta as long as corm of exopodite and distalmost one somewhat longer (seta/corm = 1.3), at opposite side there is a smaller seta similarly setulated somewhat shorter than corm (Fig. 4i). Inner portion of limb with a marginal row of eight scrapers and a slightly submarginal outer row of eight soft bilaterally setulated setae, members of both rows decreasing in length in direction to gnathobase. Scraper 1 shorter than distance between it and scraper 8. Scrapers biarticulate, 1 to 3 unilaterally setulated (Fig. 3i), from scraper 4 to 8 setules are progressively replaced by spinules and later by denticles (Fig. 4 j–4m). Between scraper 8 and gnathobase there is one short seta with unilateral row of short setules and close to base of scraper 4 there is one small sensillum (Fig. 4k, arrow). Distal part of gnathobase with four elements: 1 stick conical sensillum, 2 and 4 subcylindrical narrowing towards a blunt apex and 3, longest of the group, similar to afore described ones but with a sort of blunt hook at tip. Filter plate with eight biarticulate setae (Fig. 4n).
Limb III (Fig. 5a–5b). Epipodite large with a finger-like distal part as long as 1.4 length of exopodite. Exopodite subretangular about twice longer than wide with longitudinal row of long setules at both lateral sides and a transverse row at proximal part of inner side. With eight setae, three bilaterally setulated lateral seta and five distal setae. Seta 3 (counting from epipodite to endite) shorter than 1 and 2, as long as 1.6–1.9 distal width of corm. Setae 8 longest apical seta, 5 and 6 short, 4 and 7 relatively long but shorter than 8; seta 5 shortest of exopodite, as long as or shorter than with of segment; seta 4 2.2–3 times longer than 5 (Fig. 5a). Endite five with one long stiff anterior seta with rows of short setulae (Fig. 5b, 1) and one posterior soft seta bilaterally setulated. Endite four with one anterior naked seta (Fig. 5b, 2), one posterior seta similar to that of endite five and one small sensillum at base of anterior seta (Fig. 5b, arrow). Endite three with one anterior seta (Fig. 5b, 3) and two posterior seta similar to those describe in endite four, with a small sensillun at base of anterior seta (Fig. 5a, arrow). Proximal endite with a row of four anterior setae bilaterally setulated and four posterior setae with blunt tip and one short sensillum at proximal end row, two distlamost setae with unilateral row of short setules at distal part. Gnathobase with a proximal finger-like sensillum and three modified seta at apex. Filter plate with eight bilaterally setulated setae most of them subequal in size, distalmost seta shorter than others (Fig. 5b). Transparency and thinness of the integument made it difficult to observe several of the details of this part of the limb.
Limb IV (Fig. 5c–5d). Epipodite suboval with long finger-like projection as long as about 1.5 basal part. Exopodite subtriangular, with eight setae. Setae 1, 2, 3 large and feather-shaped similar in length, longest seta 1 as long as twice basal width of exopodite corm. Remaining seta at opposite side of the corm. Setae 4 to 7 bilaterally setulated, seta 4 longest of this group. Seta 8 as long as seta 4, sickle-shaped with basal part straight with some short setules at inner side and distal part curved and naked. Distal margin of exopodite between setae 3 and 4 with dense row of long setules (Fig. 5c). Inner distal portion of limb with two rows of setae with five elements each. Posterior setae similar between them with different bilateral setulation in proximal and distal parts. Anterior setae with different shapes and sizes, seta 1 longest of the group with unilateral row of denticles at distal part, seta 2 somewhat shorter than seta 1 and similar to it but with row of setules along all extension of seta, setae 3 and 4 “flaming setae”, short and wide, bottle-shaped with row of setules at distal part, seta 5 shortest of the row naked with blunt tip. Gnathobase with one geniculate setose seta as long as width of corm (Fig. 5d, arrow) and one naked short seta. Filter plate with eight bilaterally setulated setae.
Limb V (Fig. 5e–5f). Epipodite similar to that of IV limb. Exopodite large, subtrapezoidal with two partially separated lobes and seven bilaterally setulated setae. Main lobe with five setae, setae 1 and 2, long and feather-shaped, similar in length, seta 1 as long as twice length of corm. Setae 3,4, and 5 short, 3 apical and 4 and 5 lateral. Minor lobe with two setae, 6 and 7, similar in shape and size to 3, 4 and 5. Most of free rim of exopod with dense row of long setules (Fig. 4e). Inner distal portion of limb reduced to a round lobe partially covered long setules. Gnathobase with three elements, one long proximal seta with all length covered by dense setulation on one side and one row of long thick setules at distal part, another seta, shorter than that afore described seta, angularly bent with a unilateral row of thick stiff setulae and a finger-like sensillum between them (Fig. 4 f, arrow). Filter plate with seven bilaterally setulated setae (Fig. 5f).
Limb VI (Fig. 5g). Epipodite small, ovoid elongate. Rest of limb reduced to a big flat lob about 3.5 longer than wide with a row of long setules along apical and anterior rim and with dense row of short setules along all length of posterior rim (Fig. 5g, arrow). Size. Adult female 1.07–1.73 mm, juvenile 0.68–0.89 mm.
Ephippial female and male unknown.
Differential diagnosis. A. smirnovi sp. n. differs from A. curvirostris (O.F. Müller 1776) by: 1) valve with posterodorsal corner well defined, often expanded as short blunt protuberance; 2) dorsal side of valve practically straight in adults and somewhat concave in juveniles; 3) scraper 1 of thoracic limb II relatively short, shorter than distance between it and scraper 8; 4) on exopodite of thoracic limb III, seta 3 relatively long, as long as 1.6–1.9 the width of distal end of exopodite; 5) on exopodite of thoracic limb III, seta 5 short, as long as 0.8–1 the width of distal end of exopodite; 6) thoracic limb VI with dense row of short setules along all length of posterior rim.
DISCUSSION
Taxonomy. The differential diagnosis is based only on the differences found between our specimens and those from available illustration and/or descriptions from the literature that, in turn, are shared by all of them. That is, we have found differences in several characters of specimens described from North America and western Eurasia in individual comparisons but these were not included in the differential diagnosis since they also differ heterogeneously among them. This complex situation is discussed below in each case according to each part of the morphology.
Habitus. Practically all the published illustrations show that the dorsal junction point of valves is broadly rounded or an angle barely insinuated while in the Colombian specimens it is well defined and produced into a blunt protuberance. A somewhat similar posterodorsal angle was depicted, by Srámek-Hušek et al. (1962) (Fig. 113, H ), not in females but in a male specimen.
The same could be said about the carapace dorsal side, which is normally shown as a convex line in published descriptions while in this study, based on Colombian material, it is nearly straight or slightly concave in larger specimens and definitely concave in smaller ones. It is well known that in several taxa of anomopod cladocerans, mainly in Aradopoda, the dorsal outline of the carapace varies in shape depending upon the clutch size. Indeed, dorsal outline is more prominent in mature specimens which, in turn, are those of greater size. Size of the Colombian specimens, showing a dorsal margin of the valvar carapace concave, is similar to or greater than those illustrated by Smirnov (1992) and Flössner (2000), body length around 1.1 mm, with a well-convex margin. The specimen figured by Silva-Briano (1998, Fig.1) exhibits a relatively atypical habitus within the genus that differentiates it from the remaining forms described by different authors from Eurasian and American localities. The habitus, in lateral view, is grossly subtriangular, with the maximum body height at the posterodorsal angle and the posterior valvar border right-angled in shape, while in all other published illustrations, and in Colombian specimens, the maximum height is approximately in the middle of the length of the valvar carapace and the posterior border is convex in different degrees. The other existing illustration of a specimen of A. curvirostris, from North America, is that published by Birge (1918) which does not coincide with the Silva-Briano’s described form because it exhibits the habitus usually known of this species.
Armature of ventral rim of valve. Hudec (2010) shows a figure where setae are arising from a sort of inner lobes as groups of four setae (see Fig. 53 : A1 and A2). This arrangement has not been observed in our specimens and, furthermore, it has not been observed or illustrated by other authors, particularly by Fryer (1974) who provided the most detailed description of the armature of the ventral valve rim in Acantholeberis.
Antenna. We explored the possibility of using, as a discriminant feature, the density of spines on the seta arising from the basal segment of the endopod. Recently it has been shown that it is a useful trait for separating into two species the monotypic macrothricid genus Grimaldina (Neretina, Kotov, 2017). It was measured as the ratio: distance between bases of spinules/ diameter of seta. The source of data about this feature in Acantholeberis is available from published figures shown by several authors of specimens from different localities, namely: Norway, see Sars (1993, Fig. 67,1,a ), Sweden, see Lilljeborg (1900, Fig. LVII , 4), Great Britain, see Fryer (1974, Fig. 5), Russia, see Smirnov (1992, Fig. 15 ), U.S.A, see Silva-Briano (1998, Fig. 5) and Slovakia, see Hudec (2010, Fig. 53 Dx). The results obtained [author =, mean, (minimum-maximum), coefficient of variation %, (number of data)] are as follows: Sars (1993) = 3.5 (2.7–4), 20.1% (17); Liljieborg1(1900) = 2.36 (1.95–2.5), 12.2% (10); Silva-Briano (1998) = 3.15 (1.2–5.6), 43.8%, (14); Hudec (2010) = 3.21 (2.3–4.6), 21.5% (14); Fryer (1974) = 4.26 (1.5–6.5), 21.95% (20); Smirnov (1992) = 6.02 (5–8.4), 30.76% (11); present study = 3.63 (1.42–5.8), 28.22% (76). From these results it can be seen a relatively wide range of mean values of this ratio, from a 2.36 (Sweden), dense pattern of distribution, to 6.02 (Russia), spinules more sparsely distributed along the seta, and the remaining localities in between with values around 3 and 4. One-way analysis of variance indicates that variation among means is significantly greater than expected by chance, p < 0.0001 and the Tukey-Kramer multiple comparisons test shows significant differences (p < 0.001) only between Russian specimens and those from all other localities. Moreover Colombian and British specimens significantly differ (<0.05 and <0.001, respectively) from Swedish ones. The intra-sample variability of the ratio, expressed as coefficient of variation (%), is mostly high (12.2–43.8%), including the case of Colombian specimens (28.22%), for which there was a larger sample, suggests the need to operate with a high number of data. It is difficult to associate these results with any geographic or morphological pattern in a coherent scheme of taxonomic value. Therefore, this tool, which has been useful in the macrothricid genus Grimaldina, has not been shown to be effective in our case, at least in the conditions in which it was performed. Because that information comes from measurements mainly taken from figures with different levels of definition and, in most of cases, a relatively low number of “samples”, it is difficult to develop a well-supported opinion about the usefulness of this trait as a diagnostic character at the species level within this genus.
We believe that it is important to draw attention to a qualitative characteristic regarding the armature of the aforementioned seta that seems to be unique among the known descriptions and illustrations of Acantholeberis, which is the presence of denticles on the basal part of this seta in the specimens studied by Silva-Briano (1998) while the “normal” situation is that the denticles are confined to the distal segment.
Maxilla I. As far as we know, in Acantholeberis, this appendage has been described and illustrated only by Fryer (1974) where the dentate protrusion observed in our specimens is not present. The kind of maxilla I observed in our specimens resembles, in its general aspect, those described in Gondwanothrix halsei Van Damme, Shiel et Dumont 2007 and in Dumontia oregonensis Santos-Flores, Dodson 2003, also belonging to monotypic families and also considered “primitive”. This trait has not been included in the differential diagnosis because, unlike the other traits, it comes from a single comparison.
Limb II. The length of the scraper 1 of our specimens can be considered another differential feature because in the Colombian specimens this scraper is shorter than in all other known descriptions or illustrations. The relative length (measured as the ratio, length of scraper/distance between scrapers 1 to 8) are as follows: 1.2 in Sars (1993) 1.63 in Lund (1870), 1.5 in Lilljeborg (1900), 1.75 in Silva-Briano (1998), 1.62 in Hudec (2010), 0.86-0.9 in Colombian populations described herein.
Limb III. The number of setae on exopodite is another feature with unexpected variability; it differs according to author from seven to nine. Sars (1993), Smirnov (1976) and Hudec (2010), reported seven, Lund (1870), Silva-Briano (1998), Dumont, Silva-Briano (1998), Kotov (2013) and the present study reports eight as well as, Liljieborg (1900) and Smirnov (1992) found nine. Moreover there are also differences in the pattern of distribution, in the case of seven setae, they are two lateral and five apical for Sars (1993) and Smirnov (1976) but for Hudec (2010) they are three lateral and four apical. Length and shape of seta 3 of exopodite in limb III differ among them in published illustrations or descriptions by different authors from different localities. Relative length, (measured as the ratio width of corm/length of seta), is as follows: 1–1.4 Europe (Lund, 1870; Lilljeborg, 1900; Hudec, 2010); 0.5, United States of America (Silva-Briano, 1998) and 1.6–1.9 Colombia (present study). In all published illustrations and in our material this seta is feather-like, similar to almost all other setae of exopodites, but that illustrated by Silva-Briano (1998) is quite different both in length and shape, very short with the basal part bipinnate and naked at distal part. A comparison of the relative lengths of setae 4 and 5, in the illustrations of the different localities specimens shows that there are variations with possible diagnostic value: 4 as long as 5 (Lilljeborg, 1900; Lund, 1870), 4 shorter than 5 (Smirnov, 1976) and 4 longer than 5 (Silva-Briano, 1998; Hudec, 2010 and present study). However if we compare the relative length of seta 5 (measured as the ratio distal width of segment/length of setae) among European and North American populations we found that it is higher than 1.3 (mean 2.4), 1.3 in Sars (1993), 1.6 in Lund (1870), 2.3 in Lilljeborg (1900), 4.3 in Smirnov (1976), 1.2 in Hudec (2010), 1.9 in Silva-Briano (1998), and lower than 1 (0.79–0.98) in Colombian specimens.
Limb 4. Longest gnathobasic seta, geniculate and setose, in illustrations by Lund (1870), Lilljeborg (1900) and Silva-Briano (1998) and in Colombian specimens it is as long as the width of the corm but that shown by Hudec (2010) is practically twice longer.
Limb V. The number of setae of the filter plate that we observed in our material is seven, like most authors, Lund (1870), Lilljeborg (1901), Smirnov (1974), Flossner (2000), Hudec (2010) but Silva-Briano (1998) points out the presence of nine setae.
Limb VI. The known descriptions and illustrations of this appendage show a continuous row of setulae extended over the apical and outer margins with the inner margin naked, Lund (1870), Silva-Briano (1998) and Hudec (2010) or briefly extended over the extreme distal portion of the inner margin (Lilljeborg, 1900). On the other hand, in Colombian specimens the row of setulae covers the external and apical margins but there is a dense row of short spinules along the entire inner margin.
The comparative analysis of the different aspects and details of Acantholeberis morphology, in populations of different origin and its heterogeneous result regarding differences and similarities among them, has at least two possible interpretations, that it is a species with remarkable intra-population variability and morphological plasticity, a hypothesis that could not be supported by our observations, or the possibility that this genus, hitherto considered as monotypic, could be a group of taxa, species or subspecies, which until now have not been defined as such. Our study suggests that the taxonomy of this genus is much more complex and diverse than previously thought, providing evidence that it should be revised.
Biogeography. When specimens of a species are found in a place outside its recognized biogeographic range, at least two possibilities can be considered, that it is a recent introduction mainly by anthropogenic invasion or that the novelty of the finding is simply due to the lack of previous knowledge of an area insufficiently explored. Without ruling out the first alternative, we think that the present case is more likely the second alternative for the reasons discussed below. It is well known humans have greatly increased the rate of introduction of aquatic species into continental water bodies.
Means and circumstances of these deliberate or accidental introductions of a species of cladocerans or other species of small invertebrates were discussed by Havel, Shurin (2004). Taking into account the poor accessibility of the studied environments, the more likely or perhaps the only means that could have been used would be that of fish stocking, through which active specimens and propagules could be introduced through the water in which the fingerlings or juveniles were transported. In this specific case, it would be rainbow trout (Oncorhynchus mykiss Walbaum), which actually occurred in some studied bodies of water. There are at least two arguments that would not support this hypothesis. First: as it has already been pointed out, A. curvirostris and A. smirnovi sp. n. thrive in acidic waters, which would mean that the fish to be introduced, together with the cladocerans propagules, should have been bred and transported in this type of water. This is highly unlikely since the rainbow trout is generally recognized to be one of the more negatively affected salmonid by the acidity of the water (Grande et al., 1978; Graham, Wood, 1982; Weiner et al., 1986). Second, at the studied lakes, in which A. smirnovi sp. n. was present, there were no fish and in those with fish, no specimens or remains of A. smirnovi sp. n. were found. This inverse relationship can be interpreted as the possible cause of this absence of this cladoceran could have been the fish, by predation. In a previous study, it was experimentally verified (at mesocosm scale) that juveniles of O. mykiss actively preyed on limnetic cladoceran Ceriodaphnia (Herrera-Martinez et al. 2017).
We know that cladocerans are capable of generating latency mechanisms (ephippia or resting eggs) and that they can resist adverse conditions including desiccation. These characteristics are the basic conditions to permit their dispersal because they are continental organisms and therefore they live on “islands” in “dry oceans” and for jumping from one island to another they need a biotic or abiotic vector that transports them in its state of latency (Incagnone et al., 2014). For A. curvicornis, thanks to the observations by Fryer (1972) and Frenzel (1983), it is known that mictic females form an ephippium which is attached to surface of an aquatic plant. It is supposed that it remains there for some time and with decaying of the plant it will inevitably end up in the bottom sediments and that if dried it can hatch if it is rewetted (Gray, Arnott, 2012). This is one of the few cases among the Cladocera in which there is so much knowledge about what the first stage of a dispersal mechanism would be, but we know nothing about the next stage. Excluding water, as an abiotic vector, when the transfer occurs between connected water bodies, the other vectors would be the wind or terrestrial or semi-aquatic organisms with atmospheric respiration, in both cases current knowledge is very scarce. Since Darwin’s time, it has been proposed that birds would be important dispersal vectors for cladocerans, of which some cases of transport by endochory are known. It is also reasonable to suppose that mammals could also be important vectors, mainly due to exochory, for example the mud that remains in the paws or hooves of mammals when they go to drink from the shores of water bodies.
In our case, taking into account the geological history, as well as, the altitude and isolation of the water bodies where Acantholeberis was found, Panteui, Venezuela of Precambrian origin (Smirnov et al., 1995) and Andes, Colombia, the result of tectonic plate processes developed during the Mesozoic-Cenozoic (this study), it is not easy to find arguments to explain the disjunct distribution of Acantholeberis in South America through a process of vicariance. However, considering what has already been discussed about the dispersal mechanisms of these organisms, it could be assumed that the presence of Acantholeberis in South America would be due to long distance jump events mediated by migratory birds from North America, perhaps during the Neogene period. This would be another case of dispersal of an organism through a bird migration route that involves thousands of individuals and millions of years (Simpson, 1952; Pielou, 1979). Recent studies demonstrate the importance of migratory waterbirds as a dispersing agent for various microcrustaceans, particularly branchiopoda (Green et al., 2005, 2008; Sánchez et al., 2007; Viana et al., 2013). Indeed, the close relationship between the geographical distribution of Artemia with the shorebird flyways between North America and South America specifically mentioned here for Acantholeberis has been shown (Muñoz et al., 2013). The locations from where the specimens of this study were collected, in Colombia, and that of the other previous record, in Venezuela (Smirnov et al., 1995) are located on the routes of the two most important North America – South America flyways, the Central Flyway and the Atlantic Flyway (Baker, 1979; Lomolino et al., 2006; Birdlife org.) which go through the regions of North America where there is the highest concentration of records of Acantholeberis. Bird migrations, in general, would have originated in Cretaceous time (Lockley et al., 2012) but they were well developed in the Mid Miocene when at mid and high latitudes seasonality was more defined (Steadman, Martin 1984). It is known that the migration of birds North America – South America intensified after the connection of the Panama isthmus (Smith, Klicka, 2010). These two places have in common that they are high water bodies (2800–4000 masl) located in places that are very difficult to access by land and that does have acidic water. It is not known if the Acantholeberis of Venezuela belong to the same taxon that we describe from Colombia, but if it is possible that some of these sites could have acted as a dispersal source for the other or others, since it is known that these sites may be connected by migrations of birds between them (Marin, 2010). A. curvirostris lives in acidic environments because it has the necessary physiological adaptations to live in this kind of environment (Potts, Fryer, 1979) and this characteristic favors its dispersal since it limits the number of its possible competitors to other acidophilic organisms or those that have wide tolerance ranges.
It has been generally accepted that Acantholeberis has a Holarctic geographical distribution, excluding from consideration some records of occurrence in Neotropical and Oriental regions, because they were overlooked or considered doubtful identifications (Smirnov, 1976; Forró et al., 2008; Kotov, 2013). However, its more detailed pattern of distribution, within these large biogeographic regions, has not been object of much attention. The first, and only, published map of the geographical distribution of Acantholeberis was given by Smirnov (1976), this author mentioned its distribution as Holarctic but pointed out that “no record are available eastwards from the Ob basin, southwards from 40°N” (Smirnov, 1992). As regard the southern limit in Europe this assertion has been supported and confirmed by Margaritora (1985) and Alonso (1996) showing that this genus is absent from at least two of the three large peninsulas of Southern Europe. On the other hand, none of the recently published checklists on the cladoceran fauna of Eastern Asia include the presence of Acantholeberis (Klimovsky et al., 2015; Jeong et al., 2014; Kotov et al., 2011; Ji et al., 2015). Certainly, taking into account the imbalance between the number of taxonomical studies and the enormous extension of these territories a future finding of Acantholeberidae in Northern China should not be excluded (Xiang et al., 2015), but so far this has not been the case.
Notwithstanding the presence of Acantholeberis in India has been mentioned in several publications, mainly lists of species in ecological studies in general with no figures or descriptions supporting the identification, there is no certainty about the validity of such records. Sharma, Sharma (2017) in their critical revision and state-of-art review of researches about the Indian Cladocera fauna, found that there a number of dubious records and assigned those of Acantholeberis curvirostris to a list of ‘reports inquirendae’. The only published study that includes description and illustrations confirms the aforementioned doubts. Rajkumar, Hemalatha (2012) gives a brief description and some partial photographs of a Macrothricid-like cladoceran found in Bharathapuzha River, Kerala State, which is identified as Acantholeberis. However the illustrated specimen, with a Lathonura-like head, clearly does not correspond to what is usually known as this genus differing from it in several aspects of habitus, head, labrum, intestine and postabdomen, at least.
At first glance, published information about the geographic distribution of Acantholeberis in North America does not look so developed as that in Eurasia and is sometimes somewhat confused. Brooks (1959) pointed out that the distribution is “most of U.S., possibly elsewhere on continent”, in Canada this genus would be “distributed from coast to coast” (Chengalath,1982) but this assertion does not fit to shown in his Table 1 because this genus is absent in western provinces. Other authors described its distribution as more restricted: “North Central United States” (Underhill et al., 1982) and “especially in eastern Canada and New England” (Dodson et al., 2010).
In the present study, the original map (Smirnov, 1976), has been updated with the addition of more recent records (Fig. 6). This map shows that, excluding those records from South America (Smirnov et al., 1995 and present study), most of them are located indeed in North America and Eurasia but not in all the extension of these continents. They are practically constrained to northwestern Europe and eastern North America decreasing in its frequency to be completely absent in the opposite geographical direction in each continent, eastward and westward respectively and also southward in Eurasia. This geographic distribution can be interpreted as a biogeographic Euramerican pattern. The palaeocontinent Euramerica consisted in the junction of the eastern part of North America and Europe, sometimes as a group of islands, closing the space occupied by the North Atlantic. It is known that since the Mid-Late Cretaceous it was bordered at the western side by the Mid-Continental Seaway and later the Rocky Mountains, dividing longitudinally North America, and east by the Turgai Sea. This situation lasted about 25–30 Myr because the closing of the Mid-Continental Seaway in the Paleocene, in late Cretaceous also started the opening of North Atlantic ocean separating North America from Europe although a North Atlantic land bridge persisted until the Early-Eocene, allowing the biological exchange between these continents, mainly through the Thulean Bridge. At the other side of the palaeocontinent, comprised of Europe plus eastern Asia, the separation of Eastern Asia was more prolonged in time as, with a brief interruption a the northern opening in the Paleocene, it continued until the Oligocene (30 Myr) when the Tutgai Sea dried. Moreover, the uplift of the Tibet mountains, in the late Oligocene, extended the separation of eastern Asia. (Cox, 1974; Cox et al., 2016; Briggs, l987; McKenna, 1983; Tiffney, 1985; Liebherr, 1991; Tangelder, 1988; Sanmartin et al., 2001; Brikiatis, Jason, 2014).
Fig. 6.
Map of the geographical distribution of Acantholeberis. Empty circles indicate records in South America: the right one, Tepuy Maracahua, Venezuela (Smirnov et al., 1995) and the left one, the present study. Modified from Smirnov (1976) and updated with the records from the following papers: Arnott, Yan (2002); Bledzki, Rybak, (2016); Chengalath (1982); Frenzel (1983); Glagolev, Bykov (2012); Gray, Arnott, (2012); Louette et al. (2009); Shoeberg et al. (1984); Rautio (2001); Walseng et al. (2003, 2008); Ward et al. (1989); Witty (2004).
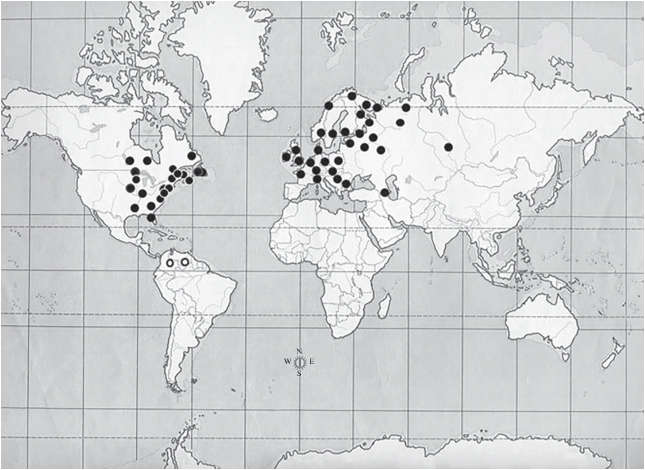
The Acantholeberidae, as well as cladocerans in general and many other organisms, were involved in massive extinctions, perhaps mainly those occurred in the late Mesozoic and early Cenozoic and later in the Neocene (Korovchinsky, 2006). There is evidence that the Anomopoda represented by more advanced forms, Aradopoda, already existed in the Mesozoic, but it is not the same with Radopoda (Van Damme, Kotov, 2016). The morphology, of Acantholeberidae, particularly of limbs, suggests that it could be considered a “primitive” Anomopod, along with (Dumontidae, Gondwanotrichidae, Eurycercidae and Ophryoxidae) with a greater antiquity within the group, originating perhaps in the Mesozoic with its important evolutionary radiation at high taxonomic levels. There is no paleontological evidence that allows us to assert when, in the geological history, Acantoleberis appeared and dispersed. However we think that its current geographical distribution analyzed in the context of geological history could reveal some clues.
According to the description of the paleogeographic history made above, in the Mid-Late Cretaceous a great epicontinental sea, Mid-Continental Seaway, began to be formed and later in the Mid Jurasic, another epicontinental sea, Turgay Seaway, appeared. Therefore Laurasia was divided into two palaeocontinents, Asiamerica and Euramerica, separated by two huge salt water biogeographical barriers. It could be assumed that perhaps during that period, which lasted 25–30 Myr, Acantholeberis or its close ancestors appeared or began the expansion of their populations. Later Euramerica, formed by the junction of western North America and Europe plus western Asia, begins to be divided by the Atlantic Ocean generating a geographic conformation which could be fitted to the current distribution of Acantholeberis. It is known that in Cretaceous – Early Cenozoic a significant mass extinction occurred whose causes, although this is still a matter of discussion, would be the impact of an asteroid, Chicxulub impact, and an extensive volcanic eruption, Deccan Traps volcanism, having as one of its main consequences the acidification of marine and continental water bodies (Archibald, Fastovsky, 2004; Bond, Grasby, 2017; Lomolino et al., 2006). Considering that Acantholeberis is capable of supporting and reproducing in acidic waters, it is possible to conjecture that the current populations would be the descendants of the survivors of this ecological catastrophe and that during the recovery stage they established and expanded their populations.
It is also interesting to point out that the biogeographic barrier constituted by the Mid-Continental Seaway was broken by the closure of the sea in the Late Cretaceous-Early Paleogene and it was partially supported by the uplift of the Rocky Mountains, and on the other hand the eastern barrier constituted by the Turgay Seaway, with a brief interruption in the Eocene, lasted until the Oligocene. The last mentioned barrier could be related, perhaps in part, to the geographic separation of phylogroups as European-Western Siberian and Eastern Siberian-Far Eastern in species of the genus Moina (Bekker et al., 2016).
Список литературы
Alonso M., 1996. Crustacea, Branchiopoda. Madrid: Museo Nacional de Ciencias Naturales. 486 p.
Archibald D., Fastovsky, D., 2004. Dinosaur extinction // The Dinosauria. 2nd Edition. Weishampel D., Dodson B., Halszka O. (Eds). Berkeley: University of California Press. P. 672–684.
Arnott S.E., Yan N.D., 2002. The influence of drought and re-acidification on zooplankton emergence from resting stages // Ecological Applications. V. 12. P. 138–153.
Baker R.R., 1979. The evolutionary ecology of animal migration. London: Hodder & Stoughton. 448 p.
Bekker E.I., Karabanov D.P., Galimov Y.R., Kotov A.A., 2016. DNA Barcoding reveals high cryptic diversity in the North Eurasian Moina species (Crustacea: Cladocera) // PLoS ONE. V. 11. e0161737. https://doi.org/10.1371/journal.pone.0161737
Bendt T., 1988. Parasiten und Epibionten der Blattfusskrebse. II. Befall durch ein- und mehrzellige Tiere // Mikrokosmos, V. 77. P. 83–91.
Birge A., 1918. Water-fleas (Cladocera). Freshwater biology. Ward H.B., Whipple G.C. New York: John Wiley & Sons. P. 676–740.
Bledzki L.A., Rybak J.I., 2016. Freshwater crustacean zooplankton of Europe: Cladocera and Copepoda (Calanoida, Cyclopoida). Key to species identification, with notes on ecology, distribution, methods and introduction to data analysis. London etc.: Springer. 923 p.
Bond D.P.G., Grasby S.E., 2017. On the causes of mass extinctions // Palaeogeography, Palaeoclimatology, Palaeoecology. V. 478. P. 3–29.
Briggs J.C., 1987. Plate tectonics // Developments in Palaeontology and Stratigraphy. V. 10. P. 1–204.
Brikiatis L., Jason A., 2014. The De Geer, Thulean and Beringia routes: key concepts for understanding early Cenozoic biogeography // Journal of Biogeography. V. 41. P. 1036–1054.
Brooks J.L., 1959. Cladocera. Ward & Whipple Fresh-Water Biology, 2nd edition. Edmondson W.T. (Ed.). New York: Wiley. P. 587–656.
Chatterjee T., Kotov A.A., Fernández-Leborans G., 2013. A checklist of epibiotic ciliates (Peritrichia and Suctoria) on the cladoceran crustaceans // Biologia. V. 68. P. 439–447.
Chengalath R., 1982. A faunistic and ecological survey of the littoral Cladocera of Canada // Canadian Journal of Zoology. V. 60. P. 2668–2682.
Cox B., 1974. Vertebrate palaeodistributional patterns and continental drift // Journal of Biogeography. V. 1. P. 75–94.
Cox C.C., Moore P.D., Ladle R.J., 2016. Biogeography: An Ecological and Evolutionary Approach, 9th Edition. New York: Wiley-Blackwell. 494 p.
Dodson S.L., Cáceres C.E., Rogers D.C., 2010. Chapter 20. Cladocera and Other Branchiopoda // Ecology and Classification of North American Freshwater Invertebrates. Third Edition. Thorp J.H., Covich A.P. (Eds). Amsterdam: Academic Press. P. 773–827.
Dumont H.J., Silva-Briano M., 1998. A reclassification of the anomopod families Macrothricidae and Chydoridae, with the creation of a new suborder, the Radopoda (Crustacea: Branchiopoda) // Hydrobiologia. V. 384. P. 119–149.
Flössner D., 2000. Die Haplopoda und Cladocera (ohne Bosminidae) Mitteleuropas. Leiden, Backhuys. 428 p.
Forró L., Korovchinsky N.M., Kotov A.A., Petrusek A., 2008. Global diversity of cladocerans (Cladocera; Crustacea) in freshwater // Hydrobiologia. V. 595. P. 177–184.
Frenzel P., 1983. The attachment of the ephippium of Acantholeberis curvirostris O. F. Müller (Cladocera: Macrothricidae) // Hydrobiologia. V. 107. P. 255–259.
Fryer G., 1972. Observation on the ephippia of certain macrothricid cladocerans // Zoological Journal of the Linnean Society. V. 51. V. 79–96.
Fryer G., 1974. Evolution and adaptive radiation in the Macrothricidae (Crustacea: Cladocera): a study of comparative functional morphology and ecology // Philosophical Transactions of the Royal Society London. Series B. V. 269. P. 137–274.
Fryer G., 1995. Phylogeny and adaptive radiation within the Anomopoda: a preliminary exploration // Hydrobiologia. V. 307. P. 57–68.
Glagolev S.M., Bykov Y.S., 2012. Cladocerans of the rock pool on White Sea islands: no regional differences and inverse island biogeography // Arthropoda Selecta. V. 21. P. 137–148.
Gray D.K., Arnott S.E., 2012. The interplay between environmental conditions and Allee effects during the recovery of stressed zooplankton communities // Ecological Applications. V. 21. P. 2652–2663.
Graham M.S., Wood C.M., 1982. Toxicity of environmental acid to the rainbow trout: interactions of water hardness and environmental acidity // Canadian Journal of Zoology. V. 59. P. 1518–1526.
Grande M., Muniz T.P., Andersen, J., 1978. The relative tolerance of some salmonids to acid water // Verhandlungen der Internationalen Verein Limnologie. V. 20. P. 2076–2084.
Green A.J., Jenkins K.M., Bell D., Morris P.J., Kingsford R.T., 2008. The potential role of waterbirds in dispersing invertebrates and plants in arid Australia // Freshwater Biology. V. 53. P. 380-392.
Green A.J., Sánchez M.I., Amat F., Figuerola J., Hontoria F., Ruiz O., Hortas F., 2005. Dispersal of invasive and native brine shrimps Artemia (Anostraca) via waterbirds // Limnology and Oceanography. V. 5. P. 737–742.
Havel J.E., Shurin J.B., 2004. Mechanisms, effects, and scales of dispersal in freshwater zooplankton // Limnology and Oceanography. V. 49. P. 1229–1238.
Herrera-Martínez Y., Paggi J.C., Garcia C.B., 2017. Cascading effect of exotic fish fry on plankton community in a tropical Andean high mountain lake: a mesocosm experiment // Journal of Limnology. V. 76. P. 397–408.
Hudec I., 2010. Anomopoda, Ctenopoda, Haplopoda, Onychopoda (Crustacea: Branchiopoda). Fauna Slovenska. V. 3. Bratislava: Vydavateľstvo SAV. 496 p.
Incagnone G., Marrone F., Barone R., Robba L., Naselli-Flores L., 2014. How do freshwater organisms cross the “dry ocean”? A review on passive dispersal and colonization processes with a special focus on temporary ponds // Hydrobiologia. V. 750. P. 103–123.
Jeong H.G., Kotov A.A., Lee W., 2014. Checklist of the freshwater Cladocera (Crustacea: Branchiopoda) of South Korea // Proceedings of the Biological Society of Washington. V. 127. P. 216–228.
Ji G. H., Xiang X.F., Chen S.Z., Yu G.L., Kotov A.A., Dumont H.J., 2015. Check-list of Chinese Cladocera (Crustacea: Branchiopoda). Part II. Order Anomopoda (families Macrotrichidae, Eurycercidae and Chydoridae) // Zootaxa. V. 4044. P. 241–269.
Klimovsky A. I., Bekker E.I., Sinev A.Y., Korovchinsky N.M., Smirnov N.N., Kotov A.A. 2015. Cladocera (Crustacea,Branchiopoda) of Central Yakutia. 4. Taxonomic faunal and zoogeographical analyses // Zoologicheskiy Journal. V. 94. P. 1367–1378. [in Russian]
Korovchinsky N.M., 2006.The Cladocera (Crustacea: Branchiopoda) as a relict group // Zoological Journal of the Linnean Society. V. 147. P. 109–124.
Kotov A.A., 2013. Morphology and phylogeny of the Anomopoda (Crustacea: Cladocera). Moscow: KMK Scientific Publishers. 650 p. [in Russian]
Kotov A.A., 2016. Faunistic complexes of the Cladocera (Crustacea, Branchiopoda) of Eastern Siberia and the Far East of Russia // Zoologicheskiy Zhurnal. V. 95. P. 748–768. [in Russian]
Kotov A.A., Forró L., Korovchinsky N.M., Petrusek A., 2013. World checklist of freshwater Cladocera species. World Wide Web electronic publication. Available from: http://fada.biodiversity.be/ group/show/17 [last consulted on 2020/03/19]
Kotov A.A., Korovchinsky N.M., Sinev A.Y., Smirnov N.N., 2011. Cladocera (Crustacea, Branchiopoda) from the Zeya river Basin (Amur Region, Russian Federation). 3. Systematic faunistic and zoogeographical analyses // Zoologicheskiy Zhurnal. V. 90. P. 131–142. [in Russian]
Liebherr J.K., 1991. Phylogeny and revision of the Anchonemus clade: the genera Tetraleucus, Anchonemus, Sericoda and Elliptoleus (Coleoptera: Carabidae: Platynini) // Bulletin American Museum of Natural History. V. 202. P. 1–163.
Lilljeborg W., 1900. Cladocera Sueciae // Nova Acta Regiae Societatis Scientiarum Upsaleinsis, Seriei Tertiae. V. 19. P. 1–701.
Lockley M.G., Lim J.D., Kim J.Y., Kim K.S., Huh M., Hwang K.G., 2012. Tracking Korea’s early birds: a review of Cretaceous avian ichnology and its implications for evolution and behavior // Ichnos. V. 19. P. 17–27.
Lomolino M.V., Riddle B.R., Whittaker R.J., 2006. Biogeography. Fifth Edition. Oxford: Oxford University Press. 845 p.
Louette G., Declerck S., Vandekerkhove J., Meester L., 2009. Evaluation of restoration measures in a shallow lake through a comparison of present day zooplankton communities with historical samples // Restoration Ecology. V. 17. P. 629–640.
Lund L., 1870. Bidrag til cladocernes morphologie og systematic // Naturhistorisk Tidsskrift. V. 3. P. 129–174.
Margaritora F.G., 1985. Cladocera. Fauna d’ltalia. V. 23. Bologna: Edizioni Calderini. 399 p.
Marin G., 2010. Acerca del origen y biogeografía de la avifauna del pantepuy o Croizat versus Mayr: una revisión crítica // The Biologist. V. 8. P. 79–108.
McKenna M.C., 1983. Cenozoic paleogeography of North Atlantic land bridges // Structure and development of the Greenland-Scotland bridge: New concepts and Methods. Bott M.H.P., Saxov S., Talwani M., Thiede J. (Eds). New York: Plenum. P. 351–395.
Muñoz J., Amat F., Green A.J., Figuerola J., Gómez A., 2013. Bird migratory flyways influence the phylogeography of the invasive brine shrimp Artemia franciscana in its native American range // Peer J. V. 200. P. 1–28.
Nenniger U., 1948. Die Paritrichen der Umgebung von Erlangen mit besonderer Beruchsichtigung ihrer Wirtspexifität // Zoologische Jahrbücher. V. 77. P. 169–266.
Neretina A. N., Kotov A.A., 2017. Old World-New World differentiation of so-called “circumtropical” taxa: the case of rare genus Grimaldina Richard, 1892 (Branchiopoda: Cladocera: Macrothricidae) // Zootaxa. V. 4291. P. 295–323.
Pielou E.C., 1979. Biogeography. Wiley Intersc. Publ. 351 p.
Potts W.T.W., Fryer G., 1979. The Effects of pH and Salt Content on Sodium Balance in Daphnia magna and Acantholeberis curvirostris (Crustacea: Cladocera) // Journal of Comparative Physiology. V. 29. P. 289–294.
Rajkumar R., Hemalatha K.K., 2012. Occurrence of the genus Acantholeberis (Crustacea; Branchiopoda; Cladocera; Macrothricidae) in Bharathapuzha River, Palakkad, Kerala State, India // Millennium Zoology. V. 13. P. 5–8.
Rautio M., 2001. Ecology of zooplankton in subarctic ponds, with a focus on responses to ultraviolet radiation // Kilpisjärvi Notes. V. 15. P. 1−30.
Sánchez M.I., Green A.J., Amat F., Castellanos E.M., 2007. Transport of brine shrimps via the digestive system of migratory waders: dispersal probabilities depend on diet and season // Marine Biology. V. 151. P. 1407–1415.
Sanmartín I., Enghoff H., Ronquist F., 2001. Patterns of animal dispersal, vicariance and diversification in the Holarctic // Biological Journal of the Linnean Society. V. 73. P. 345–90.
Santos-Flores C.J., Dodson S.I., 2003. Dumontia oregonensis n. fam., n. gen., n. sp., a cladoceran representing a new family of ‘Water-fleas’ (Crustacea, Anomopoda) from U.S.A., with notes on the classification of the Order Anomopoda // Hydrobiologia. V. 500. P. 145–155.
Sars G.O., 1993. Om de i Christiania’s omegen forecommenden ferskvandskredsdyr = On the freshwater Crustacea occurring in the vicinity of Christiania. Bergen: University Publisher. 197 p. [English translation of Sars’ dissertation of 1861]
Sharma B.K., Sharma S., 2017. Crustacea Branchiopoda (Cladocera) // Current status of freshwater faunal diversity in India. Kailash C., Gopi K.C., Rao D.V., Valarmathi K., J.R.B. (Eds). Kolkata: Zoological Survey of India. P. 199–223.
Shoeberg S.A., Maccubbin A.E., Hodson R.E., Cellulose digestion of freshwater crustaceans. Limnology and Oceanography. V. 29. P. 1132–1136.
Silva-Briano M., 1998. A revision of Macrothricid-like anomopods. Ph. D. Thesis, Ghent: Ghent University. 388 p.
Simpson G.G., 1952. Probabilities of dispersal in geologic time // Bulletin of the American Museum of Natural History. V. 99. P. 163–176.
Sinev A.A., Gu Y., Han B.P., 2015. Cladocera of Hainan Island, China // Zootaxa. V. 4006. P. 569–585.
Smirnov N.N., 1976. Macrothricidae and Moinidae of the World’s fauna. Fauna SSSR. New Series. V. 112. Rakoobraznye I. № 3. Leningrad: Nauka. 237 p. [Russian]
Smirnov N.N., 1992. The Macrothricidae of the world. Guides to the identification of the microinvertebrates of the Continental Waters of the world. V. 1. The Hague: SPB Academic Publishing. 143 p.
Smirnov N.N., Alvarez H., CastilloM.M., 1995. Streblocerus superserricaudatus sp. nov. from Venezuela (Macrothricidae, Anomopoda, Crustacea) // Hydrobiologia. V. 312. P. 167–170.
Smith B.T., Klicka J., 2010. The profound influence of the Late Pliocene Panamanian uplift on the exchange, diversification, and distribution of New World birds // Ecography V. 33. P. 333–342.
Šrámek-Hušek R., Strašcraba M., Brtek J., 1962. Lupenonožci-Branchiopoda. Praha: Fauna ČSSR. 472 p.
Steadman D.W., Martin P.S., 1984. Extinction of birds in the late Pleistocene of North America // Quaternary extinctions: A Prehistoric revolution. Martin P.S., Lein R.G. (Eds). Tucson: University of Arizona Press. P. 66–477.
Tangelder I.R.M., 1988. The biogeography of the Holarctic Nephrotoma dorsalis species-group (Diptera, Tipulidae) // Beaufortia. V. 38. P. 1–35.
Tiffney B.H., 1985. Perspectives on the origin of the floristic similarity between eastern Asia and eastern North America // Journal of the Arnold Arboretum. V. 66. P. 73–94.
Underhill J.C., Schmid W.D., Gilbertson D.E., 1982. Taxonomic Keys to the Common Animals of the North Central States, exclusive of the parasitic worms, terrestrial insects, and birds. 4th edition. Minneapolis: Burgess. 205 p.
Van Damme K., Shiel R.J., Dumont H.J., 2007. Notothrix halsei gen. n., sp. n., representative of a new family of freshwater cladocerans (Branchiopoda, Anomopoda) from SW Australia, with a discussion of ancestral traits and a preliminary molecular phylogeny of the order // Zoologica Scripta. V. 36. P. 465–487.
Van Damme K., Shiel R.J., Dumont H.J., 2007a. Gondwanotrichidae nom. nov. pro Nototrichidae Van Damme, Shiel & Dumont // Zoologica Scripta. V. 36. P. 623.
Van Damme K., Kotov A.A., 2016. The fossil record of the Cladocera (Crustacea: Branchiopoda): Evidence and hypotheses // Earth-Science Reviews. V. 163. P. 162–189.
Viana D.S., Santamaría L., Michot T.C., Figuerola J., 2013. Migratory strategies of waterbirds shape the continental-scale dispersal of aquatic organisms // Ecography. V. 36. P. 430–438.
Walseng B., Yan N.D., Schartau A.K., 2003. Littoral microcrustacean (Cladocera and Copepoda) indicators of acidification in Canadian Shield Lakes // Ambio. V. 32. P. 208–213.
Walseng B., Yan N.D., Trevor T.W., Pawson W., Skarpaas O., 2008. Acidity versus habitat structure as regulators of littoral microcrustacean assemblages // Freshwater Biology. V. 53. P. 290–303.
Ward J.V., Voelz N.J., Harvey J.H., 1989. Groundwater faunas as indicators of groundwater quality: the South Platte River System // Colorado Water Resources Research Institute, Completion Report. V. 150. P. 1–39.
Weiner G.S., Schreck C.B., Li H.W., 1986. Effects of low pH on reproduction of Rainbow Trout // Transaction of American Fisheries Society. V. 115. P. 74–82.
Witty L., 2004. Practical Guide to Identifying Freshwater Crustacean Zooplankton. Cooperative Freshwater Ecology Unit. Sudbury: Laurentian University. 20 p.
Xiang F.X., Ji G. H., Chen S.Z., Yu G.L., Xu L., Han B.P., Kotov A.A., Dumont H.J., 2015. Annotated Checklist of Chinese Cladocera (Crustacea: Branchiopoda). Part I. Haplopoda, Ctenopoda, Onychopoda and Anomopoda (families Daphniidae, Moinidae, Bosminidae, Ilyocryptidae) // Zootaxa. V. 3904. P. 1–27.
Zoppi de Roa E., López C., 2008. An updated checklist of inland Cladocera (Crustacea: Orders Ctenopoda and Anomopoda) from Venezuela // Zootaxa. V. 1919. P. 45–57.
Дополнительные материалы отсутствуют.
Инструменты
Зоологический журнал